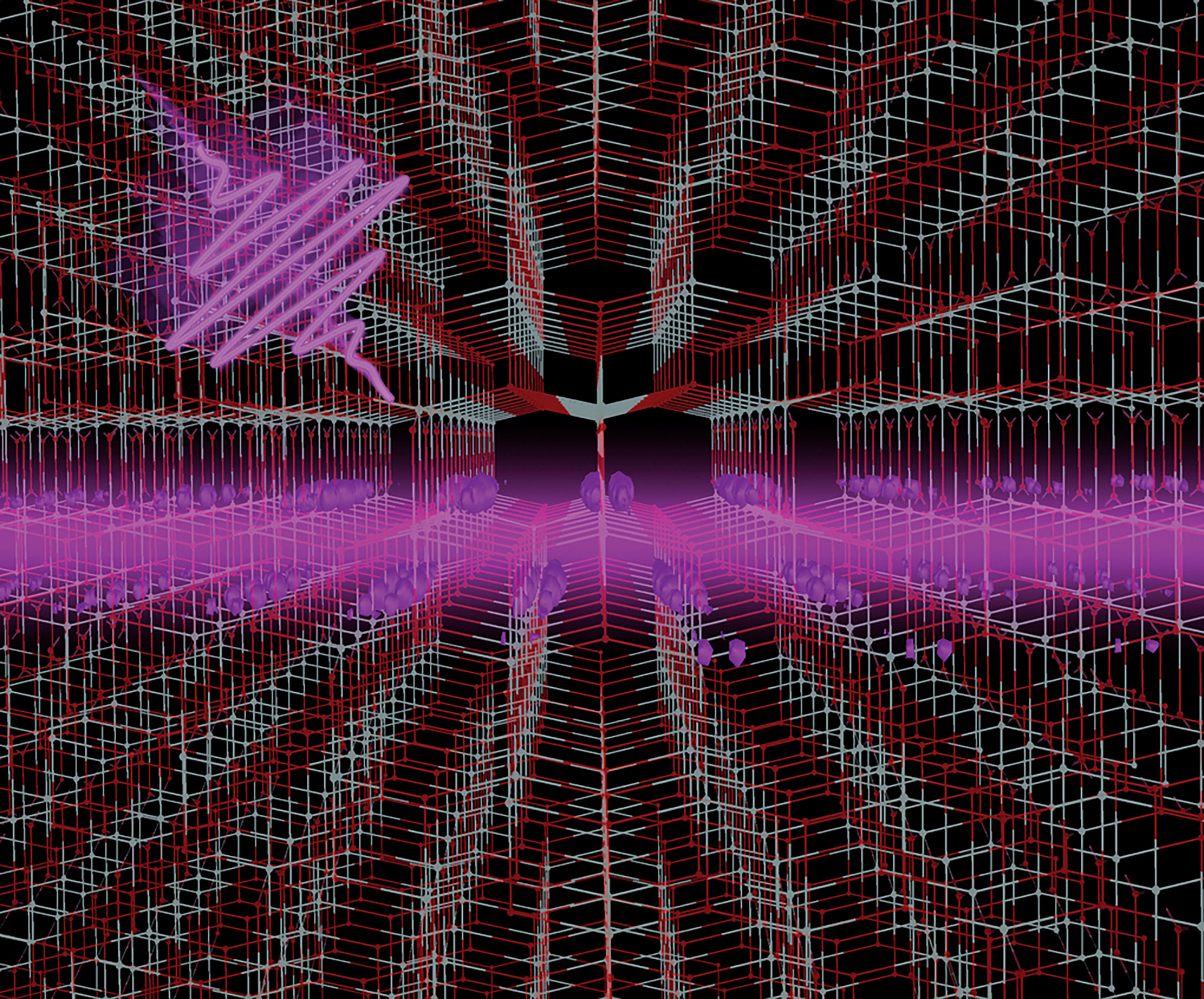
Image courtesy of Joerg M. Harms of the Max Planck Institute for the Structure and Dynamics of Matte
From early stone tools to silicon computer chips, materials have defined humankind’s progress. Electrons, discovered in 1897, are chiefly responsible for the physical properties of molecules and materials. The behavior of these charged particles determines why some metals hold an edge whereas others are pliable, why some substances react and others are inert, and why some materials conduct electricity and others insulate.
If scientists could model and predict how large numbers of electrons behave with one another, they could potentially custom-design arrangements of atoms with fantastic properties, such as high-temperature superconductivity, high-density energy storage and high-efficiency hydrogen fuel generation. They might even uncover new properties that defy current understanding.
Overcoming the complexities of the quantum world presents a daunting challenge, which a new research hub aims to meet. The Center for Computational Quantum Physics (CCQ), which launched at the Flatiron Institute in September 2017, is developing the theoretical understanding, algorithms and computational tools needed to bridge quantum mechanics and the behavior of molecules and materials.
“We are not a materials research center,” says CCQ director Antoine Georges. “That’s what makes us unique in comparison with places that apply existing methods to materials. We’re taking the problem from its foundation, from the basic computational, mathematical and physical point of view. We’re devising new concepts and new methods.”
Understanding and potentially controlling material properties is difficult because of the inconceivable number of electrons involved. A quantum system comprises all possible configurations, or states, of its particles, and the number of quantum states rises exponentially with the number of particles. A teaspoon of copper, for instance, contains more than 10 trillion electrons — that’s a lot of potential states.
The CCQ’s scientists can’t simplify the problem by extrapolating from the behavior of a single electron. In quantum physics, electrons can become entangled with one another. Once entangled, particles can no longer be treated individually, even when physically separate. The collective behavior of entangled electrons therefore drastically complicates the problem. Currently, ‘brute-force’ methods that incorporate entanglement by considering every potential interaction between every electron can handle only two or three dozen electrons at once. Many useful material properties, such as magnetism and superconductivity, only arise with millions or hundreds of millions of particles.
“There’s no way direct, brute-force simulation can work” for this problem, says Georges, who is also a professor at the Collège de France. “It’s not a matter of waiting for better computers. You’ll perhaps get an additional electron every few years, so you’re not going to get to trillions of electrons anytime soon.”
Luckily, alternatives to raw computational muscle exist. Under the leadership of Georges and center co-director Andrew Millis, also of Columbia University, the CCQ leverages computational methods to overcome the so-called ‘many electron problem’ and predict the behavior of molecules and materials. The center’s focus on developing new methods and algorithms sets it apart from research universities and government labs, Georges says. Those institutions focus on shorter-term projects, whereas algorithm and code development can take years of continued tinkering and support.
“There hasn’t been the right incentive structure in the field to keep the best programmers or algorithm developers around,” says CCQ research scientist Miles Stoudenmire. “Programming just isn’t as important elsewhere. At CCQ, it’s front and center.”
The unfulfilled need for an organization such as the CCQ helped drive its selection as the third Flatiron Institute center. The Simons Foundation had already made inroads into the field through the Simons Collaboration on the Many Electron Problem, which Millis directs, and recognized the great need for new computational methods to advance materials science.
Georges and Millis take a project-based approach to the organization of the CCQ. Some of the center’s current projects develop machine-learning methods, investigate strong light-matter coupling and build software tools. This structure gives researchers more flexibility as they navigate an uncertain path toward resolving the many electron problem, says Angel Rubio, CCQ distinguished research scientist and managing director of the Max Planck Institute for the Structure and Dynamics of Matter in Hamburg, Germany.
“If you want to be revolutionary and open new avenues, you have to tackle things that are unknown,” he says. “Of course, you have an agenda; you know what you want to solve. But in this process, you might deviate toward something that’s even more interesting than what you hoped to solve. That’s the beauty of CCQ — we’re not forcing the people to keep the originally proposed path. We’re allowing the exploring of all possibilities.”
One promising avenue for overcoming the many electron problem is the use of a mathematical concept called a tensor network. The method organizes information about a quantum system into bundles called tensors. Quantum entanglement connects the tensors into a network. For systems with a simple, regular structure, tensor networks allow researchers to approximate the system using exponentially less computational power. The approach is akin to the compression of video files, says Stoudenmire, who studies tensor networks.
Shortly after the CCQ’s launch, Stoudenmire hosted a workshop that attracted tensor network experts from around the world. The event, which included non-physicists, demonstrated that tensor networks offer unexpected potential for technologies such as artificial intelligence, he says. “Physicists have been working in this niche area developing this incredible mathematics just to solve this one tough physics problem that we have. But maybe along the way we’ve developed something more generally useful.”
Fostering collaboration through events such as the tensor network workshop is critical to the CCQ’s mission, Millis says. Events offer a chance for CCQ members to mingle with other researchers in the field — both theorists and experimentalists.
“This field needs close contact with experiment, or it becomes sterile,” Millis says. “It’s a testament to the poverty of our imaginations and the limits of our computational ability. Every few years, the field throws up a complete surprise, a class of materials that do something that nobody expected. With better theory, we’re trying to reduce the number of those surprises.”