We Finally Know Where Black Holes Get Their Magnetic Fields: Their Parents
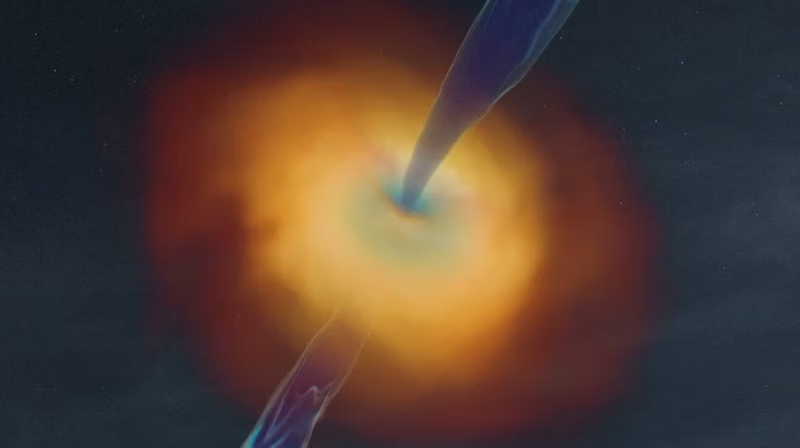
Black holes are one of the most enigmatic stellar objects. While best known for swallowing up their surroundings into a gravity pit from which nothing can escape, they can also shoot off powerful jets of charged particles, leading to explosive bursts of gamma rays that can release more energy in mere seconds than our sun will emit in its entire lifetime. For such a spectacular event to occur, a black hole needs to carry a powerful magnetic field. Where this magnetism comes from, however, has been a long-standing mystery.
Using calculations of black hole formation, scientists at the Flatiron Institute and their collaborators have finally found the origin of those magnetic fields: the collapsing parent stars of the black holes themselves. The researchers report their results November 18 in The Astrophysical Journal Letters.
Black holes can form after a star explodes as a supernova, leaving behind a dense remnant core called a proto-neutron star.
“Proto-neutron stars are the mothers of black holes in that when they collapse, a black hole is born. What we are seeing is that as this black hole forms, the proto-neutron star’s surrounding disk will essentially pin its magnetic lines to the black hole,” says Ore Gottlieb, the study’s first author and a research fellow at the Flatiron Institute’s Center for Computational Astrophysics (CCA) in New York City. “It’s very exciting to finally understand this fundamental property of black holes and how they power gamma ray bursts — the most luminous explosions in the universe.”
Gottlieb co-authored the study with fellow CCA researchers Brian Metzger, Jared Goldberg, Matteo Cantiello and Mathieu Renzo.
The Magnetism Mystery
The team initially aimed to model a star’s journey from birth to collapse to black hole formation. With their simulations, the team planned to study outflows from the black hole, like jets that generate gamma ray bursts. However, Gottlieb’s team ran into a problem with the models.
“We were not sure how to model behavior of these magnetic fields during the collapse of the neutron star to the black hole,” Gottlieb says. “So, this was a question that I started to think about for the first time.”
There were a few theories surrounding black holes and their magnetism, but none seemed to add up when accounting for the power of a black hole’s jets and gamma ray bursts.
“What had been thought to be the case is that the magnetic fields of collapsing stars are collapsing into the black hole,” says Gottlieb. “During this collapse, these magnetic field lines are made stronger as they are compressed, so the density of the magnetic fields become higher.”
The problem with that explanation was that strong magnetism in the star causes the star to lose its rotation. And without rapid rotation, a newborn black hole can’t form an accretion disk — the flow of gas, plasma, dust and particles around a black hole — and could not produce the jets and gamma ray bursts we’ve observed.
“It appears to be mutually exclusive,” says Gottlieb. “You need two things for jets to form: a strong magnetic field and an accretion disk. But a magnetic field acquired by such compression won’t form an accretion disk, and if you reduce the magnetism to the point where the disk can form, then it’s not strong enough to produce the jets.”
This meant something else was going on, and the scientists aimed to find out what it was by going straight to the source: a black hole’s parent.
A Black Hole’s Magnetic Parent: The Neutron Star
The scientists realized that perhaps previous simulations of collapsing neutron stars weren’t giving the full picture.
“Past simulations have only considered isolated neutron stars and isolated black holes, where all magnetism is lost during the collapse. However, we found that these neutron stars have accretion disks of their own, just like black holes,” says Gottlieb. “And so, the idea is that maybe an accretion disk can save the magnetic field of the neutron star. This way, a black hole will form with the same magnetic field lines that threaded the neutron star.”
The team’s calculations showed that as a neutron star collapses, before all its magnetic field is swallowed up by the newly formed black hole, the neutron star’s disk is inherited by the black hole and its magnetic field lines become anchored.
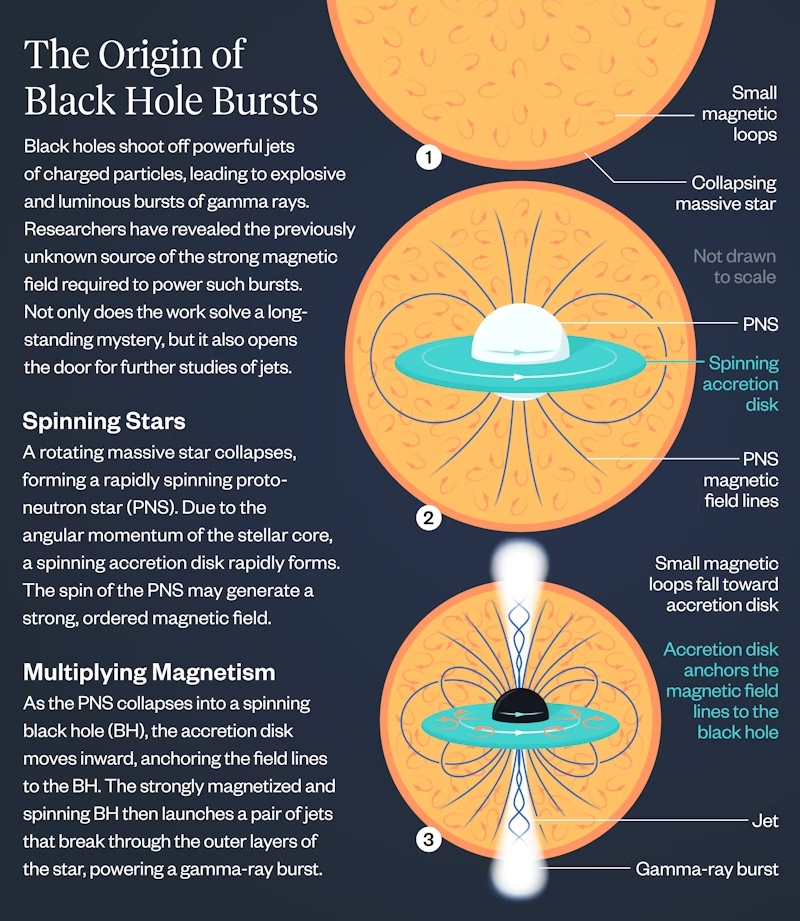
“We ran calculations for the typical values that we expect to see in these systems, and in most cases, the timescale for black hole disk formation is shorter than that of the black hole losing its magnetism,” says Gottlieb. “So the disk enables the black hole to inherit a magnetic field from its mother, the neutron star.”
Implications Across the Cosmos
Gottlieb is excited about the new discovery not only because it solves a longstanding mystery but also because it opens the door for further studies of jets.
“This study changes the way we think about what types of systems can support jet formation because if we know that accretion disks imply magnetism, then in theory, all you need is an early disk formation to power jets,” he says. “I think it would be interesting for us to rethink all of the connections between populations of stars and jet formation now that we know this.”
Gottlieb credits team science and the capabilities at CCA for making this work possible.
“This was a multidisciplinary collaboration that enabled us to address this question from different directions and form a coherent picture of a star’s evolution post-collapse,” he says. “And the generous computational resources of CCA let us run simulations of the collapse more consistently than has ever been done before. I think these two aspects led to an innovative approach.”
Information for Press
For more information, please contact Stacey Greenebaum at [email protected].