What Happened Right After the Universe Began?
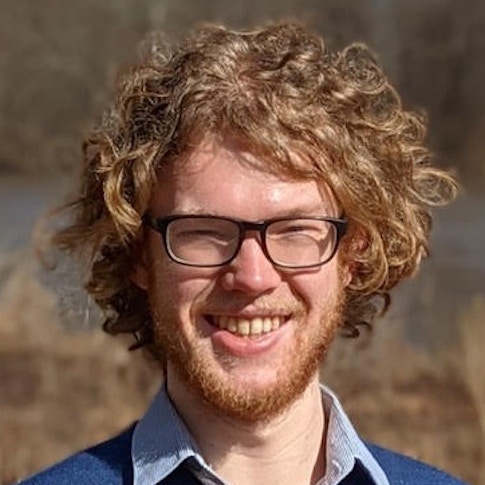
To most people, “inflation” is an economic term. But inflation also has cosmological significance. It defines the period just after the Big Bang — when the universe underwent a huge expansion and tiny fluctuations in space transformed into the seeds of the galaxies we see today. Cosmologist Oliver Philcox probes this process. By building statistical models to describe the current distribution of galaxies and radiation, he is working to tell the story of what happened billions of years ago.
Philcox just completed his first year as a Junior Fellow with the Simons Society of Fellows, working as a postdoctoral fellow with professors Lam Hui and Colin Hill in Columbia University’s High Energy Theory Group. He holds a doctorate in astrophysical sciences from Princeton University, as well as bachelor’s and master’s degrees from the University of Cambridge.
Philcox and I recently discussed his work. Our conversation has been edited for clarity.
What aspects of cosmology do you study?
How do we get a better understanding of the physical processes that happened in the earliest moments of the universe? That’s the overarching question that guides my work.
If we think of the Big Bang as time 0, what I am principally interested in is the time just after that point — up to around 10-32 seconds after the Big Bang. This is the period known as “inflation,” when the universe expanded exponentially fast. Despite its importance, inflation is still a poorly understood phenomenon, and scientists have been working for decades to understand what caused it, and why.
During inflation, the nascent universe expanded from something orders of magnitude smaller than a proton to something as big as a basketball — a huge increase. This process took only fractions of a second but set the stage for all subsequent expansion and evolution of the cosmos. It influenced the development of objects such as primordial microwave radiation, as well as galaxies that didn’t appear until hundreds of thousands, or even millions, of years later.
My approach is to start with where galaxies are positioned in the universe today, and then work backwards, deducing information about the tiny fluctuations of matter at the beginning of time and the physics that generated them. It’s very much a cosmic detective story.
What drew you to this work?
I always loved space. What kid doesn’t? At the same time, I’ve always liked solving math problems. Cosmology is a perfect marriage of these two interests. It’s allowed me to do advanced science with numerous mathematical tools to arrive at very precise results. For me, it’s an exciting area of physics, since it’s one in which traditional pen-and-paper theory is still at the heart of a lot of the major work.
Tell us more about your methods.
I work to develop theories about what happened in the early universe. The goal is to then test these theories with observational data, such as the positions of galaxies as measured by giant telescopic surveys. In some ways, this is like the particle physics experiments happening at CERN; while you can’t see what’s actually going on in the experiment, you can see the experiments’ effects — what’s left over. In my cosmology work, one can liken particle colliders to the universe, and the “leftovers” are everything in the universe we see today.
I primarily develop these theories by looking at catalogs of galaxies through the lens of statistics. Traditionally, cosmologists might look at pairs of galaxies to determine simple properties, such as the average distance between galaxies, or, more generally, the distribution of those distances across large scales. This information gives us clues about the physics and the shifts in matter that led to those galaxies, and it has led to significant improvements in our scientific understanding.
Over the last few decades, cosmologists have tried to go beyond this, to learn more by considering the statistics of three or four galaxies at a time rather than just two. This was my focus at Princeton and at the nearby Institute for Advanced Study. We’ve now reached the exciting point where we can make use of this information to learn new things about the universe.
Cosmologists with a bent toward machine learning might run these sorts of calculations on a million galaxies at once, which is now possible, albeit with some simplifying assumptions. But I prefer to work with more traditional methods, looking in depth at the relationship between just small numbers of galaxies to theorize about how they formed. I’d say these two approaches — traditional and machine learning — are highly complementary.
What did you discover during your doctoral work?
We learned a lot of interesting things about inflation, and we’ve gained insight into questions such as: How many particles were likely to have been involved in the universe’s earliest expansion? All this has been done by studying the statistics of triplets and quadruplets of galaxies.
Perhaps my most surprising finding during my doctoral research was the suggestion that, if you were to flip a set of galaxies, say from a left-to-right orientation to right-to-left, the resulting arrangement is not a mirror image of the original. This asymmetry isn’t expected in standard models of the universe, and, if true, it would have important implications for our understanding of cosmology and the birth of the universe. This finding was covered in Quanta, an editorially independent publication supported by the Simons Foundation. While these initial results were exciting, and garnered much attention, later comparisons with other datasets have suggested my findings may not hold up to scrutiny — a reminder that new and unexpected results should always be treated with caution.
What are you working on today?
At Columbia, my main focus has shifted from galaxies to the cosmic microwave background radiation, or CMB. CMB are radio wave emissions that arose some 380,000 years after the Big Bang and that today fill all space in the observable universe. Just like galaxies, CMB can be used to trace the physics of inflation; however, since the radiation comes from much earlier in cosmic history and was emitted long before galaxies were formed, it’s a much cleaner probe.
Essentially, the goal of my research is to re-create particle physics in the very early universe, as inflation was happening. This is an analogue to what’s happening at CERN, but on scales many trillions of times larger. My role is both to develop theories of what happened, and to test them with existing and new data.
Finally, what are your thoughts about the Simons Junior Fellowship?
The Junior Fellowship has provided me with total academic freedom. I have the time to explore some of the most complex and challenging questions about the universe and the physical world. I also enjoy spending time at the Simons Foundation -— I’m there three days a week — where I can meet and chat with other cosmologists, as well as researchers across many areas of computational sciences and mathematics. Being a part of this community has without a doubt enriched my thinking and my work.