What Really Happens When Stars Form?
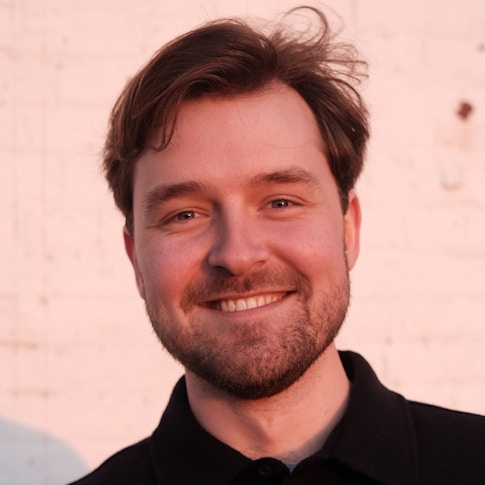
Like many of us, Lachlan Lancaster looks at the night sky with an appreciation for its beauty and mystery. But unlike most of us, he also tries to understand it. An astrophysicist at Columbia University, he works to understand how all those stars in the night sky actually came to be. Over the decades, researchers have gained important knowledge about this process, but key questions remain. And as he works to shed light on the complexities of star formation, Lancaster is also challenging conventional wisdom.
Lancaster is a first-year Junior Fellow with the Simons Society of Fellows, working with Greg Bryan in Columbia’s astronomy department. He holds a Ph.D. in astrophysics from Princeton University and earned his bachelor’s degree in physics with a specialty in astrophysics from Carnegie Mellon University.
Lancaster and I recently spoke about his work and its implications for the field. Our conversation has been edited for clarity.
What inspired your interest in astrophysics?
When I was 11 or 12, I watched the PBS special The Elegant Universe, based on the book of the same name by Brian Greene (who incidentally is a professor at Columbia). I was awed by Greene’s presentation of the beauty and complexity of the cosmos. Around that time in my life, I had already developed an interest in physics, but I was not focused on a career in astronomy — at least not yet.
Fast forward to Carnegie Mellon. When I began my undergraduate studies, I was a dual major in philosophy and physics. And while my studies were not astrophysics-focused per se, I was still interested in tackling fundamental questions about the nature of the universe. As I continued my studies, I had an exciting opportunity to conduct astrophysics research with my undergraduate adviser Matthew Walker. This is when I gained an understanding of the complex problems that astronomers like Dr. Walker were working to solve, and when my interest and passion for astrophysics became fully realized.
I think a lot of physicists, myself included, are inspired to do physics out of an interest in the fundamental laws of the universe. We are often therefore drawn to research in high-energy physics theory — string theory is a prime example of a vibrant area. For me, this branch of physics can be a bit frustrating because it involves developing theories that are very hard to test. Today, astrophysicists have powerful telescopes, as well as complex simulations, which allow us to test our theories in so many different and exciting ways.
Please describe your doctoral work.
At Princeton I studied how very massive stars — which are 10 to 100 times the mass of our sun and perhaps a million times as bright — can impact how neighboring stars form.
Broadly speaking, stars emerge when giant clouds of gas and dust collapse in on themselves over time due to gravity. When a massive star forms within one of these clouds, it is so bright that it can sometimes blow apart the gas cloud from which it originated.
With this gas cloud dispersed, other stars are less likely to form in the future, creating a kind of negative feedback loop. Understanding the details of how that feedback loop works is a very complex and exciting challenge. In fact, many of the most arresting images from NASA’s James Webb Space Telescope (JWST) capture snapshots of this process.
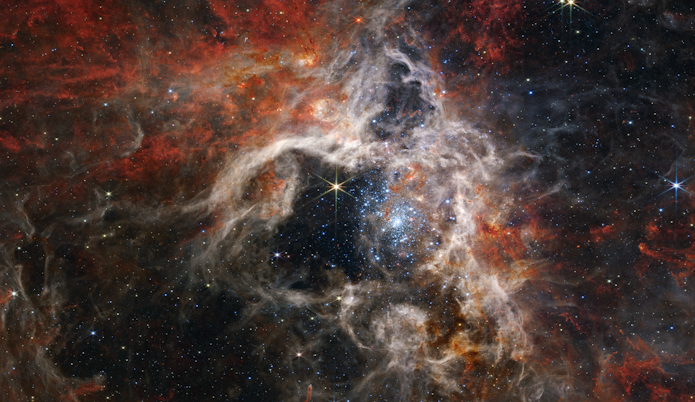
One of the most important processes driving this feedback loop are so-called stellar winds, which were the main focus of my work at Princeton. These winds arise when the stars are so bright that they end up shedding their own atmospheres. For 40 years or so astronomers have been using a spherical, uniform model to map how stellar winds expel a star’s outer layers. But as we can see from the JWST images, star-forming regions are not spherical — they are very messy.
The astronomers who came up with the original model had to make a lot of simplifying assumptions, in large part because they didn’t have the necessary computational tools to test these assumptions. Now we can use both powerful telescopes and complex simulations in concert to gather more data about these star-forming regions. These data can help us develop better assumptions that allow us to build more complicated models. For my thesis, I set out to develop a new theory of how stellar winds affect their surroundings, one that takes into account the actual messiness of star-forming regions.
What did you find?
When things are messy, stuff tends to get mixed together. And in the case of stellar winds, this can have drastic consequences. Wind moves extraordinarily fast when it comes off the star, on the order of thousands of kilometers per second. When this wind hits the surrounding gas cloud, it goes through a process called shock heating, making it about 10 million kelvins — a thousand times hotter than the surface of the sun. How hot this wind gets is critical for how efficiently it can push on surrounding gas and disperse the cloud.
Interestingly, the gas bordering these stellar winds is much cooler at only 100 kelvins, the equivalent of a frigid minus 173 degrees Celsius. Since everything in this system is so messy, the super hot gas from the stellar winds mixes with this super cold surrounding gas, causing its temperature to shift upward to a balmy 10,000 kelvins. But gas at this temperature ends up emitting wavelengths of light that escape the system. This removes energy from the system, cooling everything down after a short time.
In my work, I ran simulations demonstrating that this cooling can drastically decrease the power of stellar winds. On top of this, I built on recent work on so-called fractal mixing layers by developing a model for how this mixing and cooling happens.
Is this a new idea for the field?
Yes, in the sense that it overturns the prior spherical model. But I have the benefit of more powerful telescopes and computational tools than earlier generations of astrophysicists, so I don’t have to make as many assumptions. And I could be wrong too! In the end my work is a theory that could always be disproven by new research. In fact, at Columbia I am now trying to challenge myself, to discover if what I proposed at Princeton still holds up.
How so?
My Princeton model didn’t include two additional factors that we know are part of star formation: ionizing radiation, which works to heat up and smooth out the background cold gas so that it becomes more uniform, and strong magnetic fields that serve as a kind of rubber band that holds the gas together, helping to prevent mixing of different parts of the gas.
I added these two factors to my original simulations and models to see if it changes the picture. Thus far it hasn’t; the simulations I’m developing at Columbia look like what I developed before, which surprises me. But it’s still early days, so that picture may change.
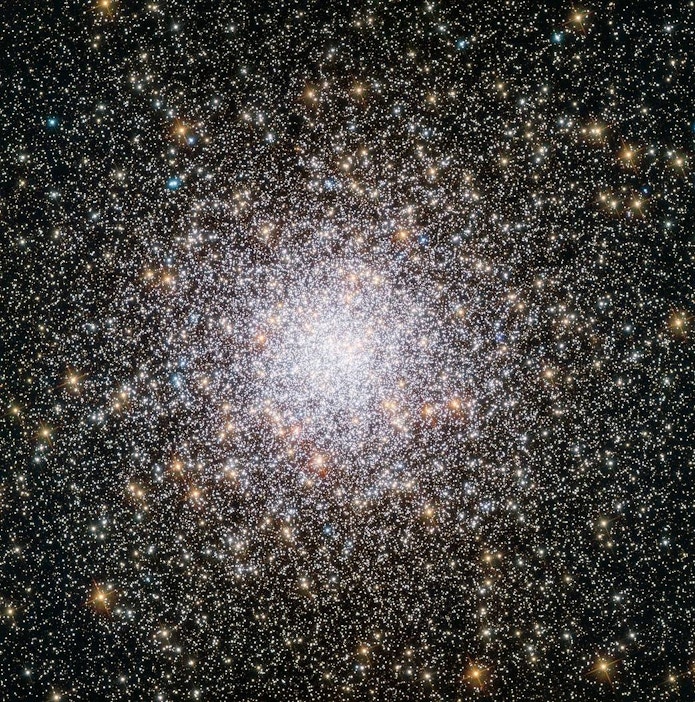
What are your longer-term goals?
Despite my recent focus on stellar winds, I was first inspired to work on star formation by objects called globular star clusters. These clusters of stars are extremely dense and very old; they formed very early in the history of the universe. We’ve known about globular star clusters for almost 250 years, but we still don’t know how they form. One of my long-term goals is to help uncover the mystery of where these star clusters come from.
Finally, what are your thoughts about the Simons Junior Fellowship?
I’m still in the first year and getting used to the reality of developing my own research program, which is new and different from the structure of graduate school.
Given that new reality, the community that comes from within the Simons Society of Fellows is very welcome. It brings together people from so many different fields in a way that’s unique and hard to replicate organically. Another positive aspect is that I know my research at Columbia is fully supported for three years, allowing me to focus entirely on my research questions — for which I am truly grateful.