Quarks, Wormholes and a New Understanding of the Physical World
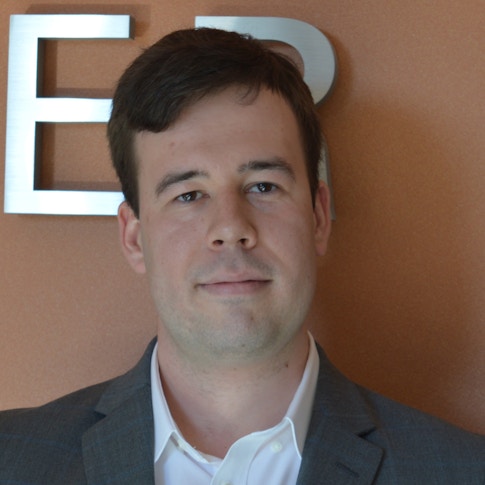
In his series Foundation, science fiction author Isaac Asimov put forth a provocative idea that, while we can never predict the behavior of any one person, once we gather enough people together, we can make accurate predictions about their group dynamics. Similarly, theoretical physicist Fedor Popov studies the behavior of large numbers of interacting quantum particles, to develop new understanding of the underlying dynamics of the physical world. In recent decades, scientists have gained significant knowledge about subatomic particles such as quarks and leptons, but much remains unknown. For example, there are still questions about how quarks interact with one another to form larger particles like protons and neutrons. But by studying the interactions of large numbers of these particles, Popov seeks to develop theoretical models — what he calls ‘toy’ models — that open up new and promising analytical possibilities.
Popov is a second year Junior Fellow with the Simons Society of Fellows, working at New York University (NYU) with Sergei Dubovsky. He completed his doctorate at Princeton University under the supervision of Igor R. Klebanov, and his undergraduate and master’s degrees at the Moscow Institute for Physics and Technology, working primarily with Emil T. Akhmedov.
Popov and I recently discussed why his work. Our conversation has been edited for clarity.
What drew you to theoretical physics?
One aspect of theoretical physics that fascinates me is the ‘least idea principle’: the notion of employing the minimum possible effort to achieve a task. Humans often employ this principle in our daily lives; it’s why we may choose to take the elevator versus climb several flights of stairs. But it turns out that is also true for any physical system no matter the size — from subatomic particles to large-scale gravitational interactions.
To the best of our knowledge, particles within an atom also try to do their work as efficiently as possible. And while we don’t yet know precisely what happens far below the subatomic level — at the level of individual quarks, for example — we can try to craft fundamental principles by developing what I call ‘toy’ models to ultimately understand why the physical world is like it is. It’s these fundamental principles that, to me, showcase the underlying beauty of the universe.
One tool you use to construct these toy models is called a large-N model. What is it?
I want to understand the interactions taking place within atoms, and the Standard Model of particle physics, developed in the 1970s, has already revealed much about these interactions. For example, we know that quarks are the building blocks of protons, which in turn are a main component of atoms. The Standard Model has also shown that, interestingly, a single quark is never found on its own. Instead, quarks are generally bound together in twos or threes — and efforts to tear them apart only serve to strengthen their bond.
This phenomenon, called ‘confinement’, has been puzzling physicists for more than 50 years, and is the focus of the Simons Collaboration on Confinement and QCD Strings, established in 2022 and led by Dr. Klebanov, my doctoral advisor at Princeton.
It would be incredibly challenging to gain insight into the phenomenon of confinement through the study of individual quarks. By examining how large numbers of quarks interact with each other, however, we can create models that are better equipped to explain current experimental results.
We call these large-N models, and that’s what I study. One of my achievements at Princeton was the discovery of a new class of large-N models that showed how quarks would behave if they were tensors, meaning a quantity with three separate labels that allow for distinguishing each component. By considering this special interaction, we found that such models could describe quite important physical phenomena like superfluidity or turbulence.
Tell us more about your doctoral work. What else did you investigate?
Princeton was a great place because so many people were exploring fascinating questions about particle physics. One project during that time centered on Einstein-Rosen bridges, more commonly known as wormholes. Popular in science fiction but still purely theoretical, wormholes are structures that can connect two distant points by bending the fabric of space-time
At Princeton, Juan Maldacena, Alexey Milekhin and I showed that two magnetically charged black holes that rotate around each other could, theoretically, interact in such a way to form a wormhole, though our results indicated that the time to travel from point A to point B via this wormhole was slower than traditional methods of travel. Despite this result, I am still fascinated by wormholes — both the idea of them and the notion that someday they could move from the realm of science fiction to reality.
How have your interests shifted since coming to NYU for your Junior Fellowship?
I truly am interested in everything, everywhere, all at once; every aspect of theoretical physics fascinates me, which is why I’ll never have a shortage of things to study.
One current interest of mine is thermalization, or information loss. We know that as time passes the information we transmit digitally will be lost — hard drives eventually break down, for example. I liken it to the way seashells erode over time, breaking down to eventually become grains of sand. That return to sand is one way to think about information loss.
A proposed alternative to traditional information computing involves quantum mechanics, though that comes with a whole series of challenges that are currently being explored. For example, can we preserve information across different quantum states in order to prevent its destruction — and how do we do it? In 2022, we proposed a possible way to separate different parts of a quantum state from each other so they can’t interact, which can in turn protect information from getting lost. It is like putting a shell in a protective box to protect it from decay.
Finally, what are your thoughts about the Simons Junior Fellowship?
The Junior Fellowship provides an opportunity that I may not have otherwise: to interact with so many people from different fields within and beyond physics, learn about their research and see how their work could be applied to my own.
For example, once at the Simons Foundation I happened to run into Michele Fornea, another Junior Fellow who is a mathematician and number theorist. Michele was describing how to estimate an integral, which was important in number theory, and I was able to share with him how the integral has a quite profound meaning for my own study of theoretical physics. It was a lovely example of how different fields can connect and inform each other.