
Jumpy Genes in the Aging Brain
The human genome contains a startling amount of so-called parasitic DNA, repetitive genetic sequences that can copy themselves and jump from place to place. Known as transposable elements, or transposons, these virus-like sequences make up almost 50 percent of our DNA.
Transposons have been both a boon and a hindrance to their hosts. In evolutionary terms, mobile elements have likely played a helpful role by boosting genetic diversity over time. Their replication and absorption into the genome offers reams of new material that organisms have co-opted for development, immunity and other functions. But if transposons replicate and implant themselves within an important gene, disrupting its function, they can wreak havoc on cells and tissues. One class of transposable elements, known as retrotransposons, have been linked to a number of diseases in humans, including hemophilia, muscular dystrophy and cancer.
Given transposons’ potential for damage, cells have developed defensive mechanisms to keep them in check. But growing evidence suggests that these mechanisms falter with age, and that retrotransposon activity could contribute to age-related decline. In the last few years, researchers have shown that active mobile elements not only disrupt DNA but can also incite the cell’s immune defenses and trigger inflammation, a common culprit in aging. “The idea that transposable elements are recognized by the innate immune system has gained huge traction,” says John Sedivy, director of the Center for the Biology of Aging at Brown University, in Providence, Rhode Island. What’s more, work by Sedivy and others has shown that drugs that block retrotransposon activity can slow these ill effects in animal models. Several such drugs approved for other uses are already in clinical trials for various different diseases.
Researchers are now exploring the specific role that retrotransposons play in the aging brain. Early evidence suggests that brain cells may be particularly vulnerable to mobile elements, and retrotransposon activity has been tied to different types of neurodegenerative disorders, including ALS and Alzheimer’s disease. “The nervous system, for reasons we don’t understand, seems to be a particularly permissive tissue for retrotransposons,” Sedivy says. Better understanding of how retrotransposons affect the brain will require more sensitive methods for tracking mobile elements in single cells and for assessing how different cells, including neurons, astrocytes and microglia respond to retrotransposon activity.
Jumping genes in the brain
Though transposons make up an outsize portion of our genome, the vast majority exist as a sort of fossil — remnants of an evolutionary past that have been rendered inactive over time. Only a fraction remains mobile, and the types and frequency of transposons that are still capable of jumping vary widely from species to species. In humans, the predominant mobile form is known as LINE-1 or L1. Our genomes have hundreds of thousands of copies of L1, comprising about 17 percent of our DNA. Only 100 or so are highly active.
Cells have evolved multiple mechanisms for controlling mobile elements. To prevent transcription, transposon-heavy regions are packed into tightly wound regions of chromatin known as heterochromatin. Backup systems of small RNAs silence those that slip through. But those defenses aren’t foolproof. Research suggests that in humans, L1 retrotransposition occurs in the germ line in approximately 1 in 100 – 200 births. Indeed, because retrotransposon segments have been repurposed to perform useful functions, including synaptic plasticity, fully shutting them down may not be feasible. “The genome faces an impossible challenge to silence the mobile elements that are a danger without shutting off essential functions that rest upon the very similar sequences that play important roles,” says Josh Dubnau, director of the Center for Developmental Genetics at Stonybrook University in New York.
Though the bulk of research on transposable elements has focused on the germ line, we now know that transposons can also replicate in somatic tissue, with some of the earliest evidence coming from brain cells. Nearly 20 years ago, in the early days of RNA sequencing, Fred “Rusty” Gage, a neuroscientist at the Salk Institute in San Diego, and collaborators were analyzing the transcriptional differences between neural stem cells, neural progenitor cells and mature neurons. To their surprise, the vast majority of differences, particularly in neural progenitors, were mobile elements. (Nowadays, most RNA-Seq technologies filter out repetitive elements because they are so abundant.) Using a fluorescent reporter that activates when a retrotransposon has inserted into chromosomal DNA in mice, Gage and collaborators showed that mobile elements can replicate in neuronal precursor cells. Gage is now studying how different diseases and aging affect retrotransposon activity in neurons by converting fibroblasts from people of different ages directly into neurons.
A number of studies have since shown that that L1s can replicate in other tissue types, and have confirmed activity in neurons and glia, particularly in the hippocampus. Retrotransposons may even play a positive role during neural development. Gage theorizes that evolution may have co-opted retrotransposon activity to boost neural diversity. “More diversity in brain cells means we have a more diverse capacity to solve problems,” Gage says. Exactly how often retrotransposons are active in neurons is still a matter of debate in the field, in large part because detecting these events in single cells is challenging. According to Gage, current technologies for measuring novel retrotransposon insertions estimate one to two events per cell.
Though scientists haven’t conducted a comprehensive survey comparing retrotransposon activity in different types of cells, transposons do seem to be especially active in neural lineages. Scientists don’t yet know why, but Sedivy suspects it may relate to neurons’ long life span and limited capacity to renew in the adult brain. “Aside from limited parts of the brain, we have the same neurons for 70 years,” Sedivy says. “Cell division in many ways is a wonderful opportunity to get rid of cells that have been damaged, but you can’t do that in the central nervous system, which makes them vulnerable to retrotransposon activation.”
Losing control
Sedivy and collaborators first became interested in retrotransposons’ role in aging about 10 years ago, when they were studying senescence, an age-related phenomenon in which cells stop dividing. (For more on senescence and aging, see “In Senescent Cells, a Promising Route to Slowing Brain Aging.”) The researchers found that senescent cells showed broad changes in their patterns of chromatin packing. Nonpacked regions of DNA, known as euchromatin, became more closed, while heterochromatic regions, especially huge heterochromatin blocks that are full of repetitive elements, became more open, promoting transposon activity. Sedivy’s team later found the same pattern in liver and skeletal muscle in aging mice, showing that aging cells lose their ability to suppress transposons.
Subsequent research in flies suggests that the boost in transposon activity that occurs with age is a cause rather than a consequence of aging — caloric restriction, which extends life span in flies and many other species, suppresses age-related transposon activation. In worms, flies, mice and human cells, genetic manipulations that block age-related decline in heterochromatin also decrease transposon activity and extend life span.
Scientists don’t yet know exactly why aging cells seem to lose the ability to tamp down retrotransposon activity. “I think aging is essentially a failure to maintain homeostasis — the body is constantly combating entropy and wear and tear, and the process is not perfect,” Sedivy says. “Macromolecules in the body accumulate oxidative lesions with age, and homeostatic systems, such as protein turnover and DNA repair, just don’t keep up.”
Research from Sedivy’s team and others has also expanded our understanding of how mobile elements harm the cell. When they first began studying retrotransposons and aging, researchers assumed that retrotransposons’ negative effects stemmed from genome damage. But researchers have found that L1s can influence cells even without inserting into genes. Because retrotransposons resemble viruses, our innate immune system treats them like an infection. In research published in Nature in 2019, Sedivy and collaborators showed that in both senescent cells and in aging mice, replicating L1 triggered an antiviral immune mechanism called the interferon response that sparked inflammation in the tissue.
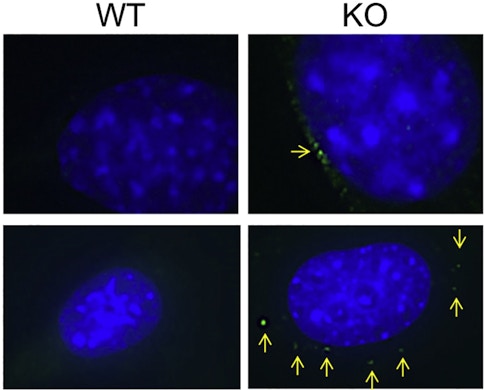
A second study from Sedivy’s collaborator Vera Gorbunova, co-director of the Aging Research Center at the University of Rochester, found a similar pattern. Gorbunova’s team had previously shown that an epigenetic regulator called SIRT6 suppresses transposons, and that mice lacking SIRT6 die prematurely. “We found that L1s go haywire in these mice, and that they have lots of inflammatory signaling in their tissue,” she says. A new study from Gorbunova’s team published in November links SIRT6 and L1 activity to human aging. The team identified a variant of the SIRT6 gene that is more common in centenarians and more effective at silencing L1. “This fits very well into the idea that high activity of transposons can be detrimental for longevity,” she says. “To live a long and healthy life, we need to silence them well.”
Sedivy, Gorbunova and collaborators hypothesize that the retrotransposon-induced interferon response is part of a broader phenomenon known as ‘inflammaging,’ or sterile aging — chronic low-level inflammation that occurs outside the context of infection. “There’s a growing recognition that inflammation may be the missing component for why some people develop disease and others don’t,” Gorbunova says. “Where does inflammation come from? L1 may provide an explanation.”
On the positive side, drugs that slow retrotransposon activity, including antiretrovirals used to treat HIV, have shown success in reversing this process in animal models. In their studies of retrotransposons and inflammation, Sedivy, Gorbunova and collaborators found that reverse transcriptase inhibitors decreased age-associated inflammation in mice and reduced health and life-span effects in SIRT6 knockouts. Clinical trials of these drugs for ALS, Alzheimer’s and other conditions are already underway.
Retrotransposons in the aging brain
In addition to their role in aging, retrotransposons have been implicated in a number of neurodegenerative disorders, particularly those that involve a protein called TDP-43, including frontotemporal dementia, ALS and Alzheimer’s disease. Transcriptomic data suggests that retrotransposons are more active in people with these diseases than in age-matched controls, as well as in fly models of disease. A growing body of research suggests that TDP-43 helps to silence retrotransposon activity in the normal brain, a function that goes awry in ALS and other disorders. “[Retrotransposon] replication causes DNA damage and probably contributes to neuropathology, at least in Drosophila models,” Dubnau says.
More recent work has linked retrotransposons to Alzheimer’s and other disorders involving misfolding of tau, a protein that helps stabilize the structure of nerve cells. Abnormal tau disrupts the molecular structure that keeps heterochromatin tightly packed, which can trigger activation of transposable elements in the brain in neurodegenerative disease, according to research from Bess Frost, a neuroscientist at the University of Texas Health Science Center in San Antonio, and collaborators. Her team has shown that retrotransposons are transcribed in brain tissue from people with Alzheimer’s disease, as well as in fly and mouse models with abnormal tau. Preliminary research from her group suggests that active retrotransposons trigger neuroinflammation, mirroring Sedivy and Gorbunova’s findings in non-neural cells. “Whenever you get activation of transposons, which are similar to exogenous viruses, it makes the brain or body think there is viral infection,” Frost says. “The link between transposons and inflammation has gained a lot of attention, and is very important when thinking about transposon activation in human aging and disease.”
Retrotransposons’ role in normal cognitive aging is less well understood, with the best evidence to date coming from flies. Dubnau and collaborators have shown that several transposable elements are active in the brain in aging flies, and that mutating a gene called Argonaute 2 (Ago2) increased transposon activity in the brain, worsened age-dependent memory decline and shortened life span. “Ago2 mutants have pretty normal memory and locomotion when young, but their brain ages fast,” Dubnau says. “They start to develop memory impairment at [a] much younger age than a fruit fly would.” New work from Frost and collaborators, published this year in Progress in Neurobiology, found that with age, transposon transcripts increase in the brain of wild-type mice.
Efforts to understand retrotransposons’ role in the aging brain have been stymied in part by technological challenges, particularly in single cells. Retrotransposon sequences are highly repetitive, making them difficult to track with typical methods. A standard L1 element, for example, is roughly 6 kilobases long. Reconstructing highly repetitive transcripts is challenging to do using standard short-read sequencing, which reads roughly 150 bases and then pastes together overlapping segments. “You can get some idea if transcription is increasing or not, but it’s only approximate, and difficult to pin to specific elements,” Sedivy says. These issues are more pronounced in single cells, which have little material to start with. “We have to figure out better ways of detecting insertions at the single-cell level,” Gage says.

Long-read sequencing technologies are helping to address these issues, but they are also very difficult to implement on a single-cell basis. Immunofluorescence methods to detect proteins involved in L1 activity offer an alternative approach that has been successfully used to study mobile elements’ role in cancer. Researchers are now starting to apply this method to neurons.
A study published in Cell Research in October using both immunofluorescence and a new method for assessing chromatin in single cells confirms that aging neurons may be especially vulnerable to retrotransposon activity. Bing Ren, a biologist at the University of California, San Diego, and collaborators compared age-related changes in chromatin in mice in different types of cells, including frontal cortex, hippocampus, heart, bone marrow and skeletal muscles. They found that brain cells showed the most age-related changes, with an opening of chromatin and increase in L1 activity. “I was surprised to see so much change in neurons associated with aging,” Ren says. In excitatory neurons specifically, areas that at younger ages are typically tightly wound up become much more open. “They were silent, buried in heterochromatin at birth, but then open up with age,” he says. Inhibitory neurons and glial cells, in contrast, did not show changes in heterochromatin. “This work provides amazing validation for a lot of conjecture,” Sedivy says. “To see differences among different cell types and ages, that’s all new information.” The next step will be to examine why these cell types are vulnerable.
This past year Sedivy, Gorbunova, Gage, Jef Boeke and others won a new grant from the National Institute on Aging to further explore how retrotransposons affect the aging brain. The researchers aim to better understand the life cycle of transposable elements in nondividing cells, particularly senescent cells and postmitotic neurons, both in culture and in mouse brains. “How are they becoming activated under relevant conditions in tissues, mouse models, organoids, IPS-derived neurons?” Sedivy says. “We are examining all of those things.”