Unlocking the Secrets of Neural Circuits and Development
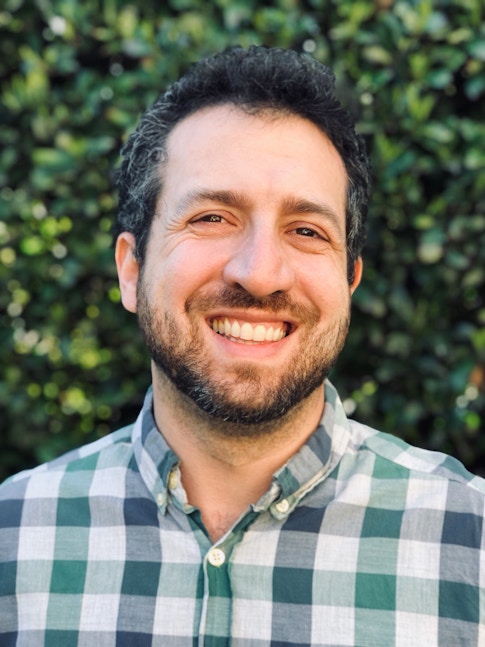
In the brain, neurons do not act alone. Instead, they form complex clusters, or circuits, that work in concert to perform a vast array of cognitive functions. Raphael (Raffi) Cohn, a Junior Fellow with the Simons Society of Fellows, is fascinated by these neural circuits and how they drive behavior. For his doctoral work in Vanessa Ruta’s lab at Rockefeller University, Cohn studied how neurons in the fruit fly brain work together to respond to different smells based on context and experience. Now, as a postdoctoral researcher with Wesley Grueber at Columbia University’s Zuckerman Institute, Cohn studies the neurons and circuits in fruit fly larvae that sense the body’s position in space, as well as sensory circuits related to touch and pain response. In his work, he examines how these neurons develop their unique identities, and their ability to guide the fly through its environment.
We recently discussed his research, including Cohn’s goal of making science more diverse. Our conversation has been edited for clarity.
What is your research focus at Columbia?
I like to think of my research as a continuing evolution in understanding neural circuits: the connections and interactions between groups of neurons that allow us to perceive and act on the world around us. When I was at Rockefeller, we studied how neural circuits enabled drosophila fruit flies to use their sense of smell to respond flexibly to a changing environment.
Today, part of my work looks further up the chain, if you will: I want to understand how neurons form in the first place. What neuronal cellular signaling and pathways are involved in this process? How do different neurons, responsible for sensing and responding to different types of stimuli, acquire their unique molecular identities and structures? I’m still working with fruit flies — fruit fly larvae specifically — which in many ways is an ideal model for addressing these questions. Not only do fruit flies have relatively few neurons compared to more complex organisms, but there are also many genetic tools available to allow neuroscientists to study their neural circuits in great detail.
A developing focus of my work involves proprioception, which is the brain’s ability to sense the position of the body in space and adapt movement accordingly. This is one of those senses that we tend not to think about too much, but it’s essential for being able to control our movement through the world; it is perhaps the most basic function of the nervous system.
Right now, I’m working on two discrete projects investigating somatosensory neurons in fruit fly larvae. The first is focused on understanding the interaction between these neurons’ genetic identity — the sets of genes they each express that control their position, shape and activity — and the molecular signals present in the developing fly’s internal environment. This is an interesting question because there are many molecular messages that are produced or diffuse throughout large areas of the fly, but each type of neuron needs to respond to these signals differently. We’re trying to unpack how this process occurs.
The next part, which I’m very excited about though it’s just getting underway, will be to dig deeper into the roles of the different types of proprioceptive and touch-sensitive neurons during animal locomotion. I will try to understand the neural circuits that use that information to adjust the animal’s movements, as well as respond to both internal and external cues. We can do this by recording the activity of these neurons in a living, moving animal and using some developmental and genetic tools to probe the neural circuits that perform this essential form of sensorimotor control.
What else are you working on?
In addition to my research projects in the lab, I also care deeply about training future scientists and helping to make science more diverse. I would like to do what I can to increase opportunities for undergraduates from diverse backgrounds who are interested in biology or neuroscience. Part of the reason I joined the Grueber lab is that it has a strong track record of supporting diversity in neuroscience, so I’ve been really happy to join in and work toward this important goal.
That sounds very rewarding. Thinking back to your time at Rockefeller, you focused on neurons responsible for olfaction, our sense of smell. Please tell us more about that.
As I mentioned, we already knew a lot about the sensory pathways that sense and process smells in the fruit fly. Indeed, previous research has established that a brain chemical called dopamine helps the fly learn to associate odors with good or bad outcomes. So if you get rid of dopamine neurons, flies can still smell and respond to odors that are innately meaningful to them, but they can no longer learn new associations. However, what was still unknown was how this occurs. During my doctoral work in the Ruta lab I worked to try to solve this puzzle. We recreated the learning experience in flies while recording what happens at the connections between the neurons that control the fly’s behavioral response to an odor. When a fly experiences a reward or a punishment, dopamine is released in specific parts of the circuit. If we present a specific odor at the same time as the dopamine is released, we can observe changes to the strength of specific connections in the animal’s neural circuitry — thereby revealing how the same sensory input can lead to different behaviors after learning. We published this work in Cell in 2015.
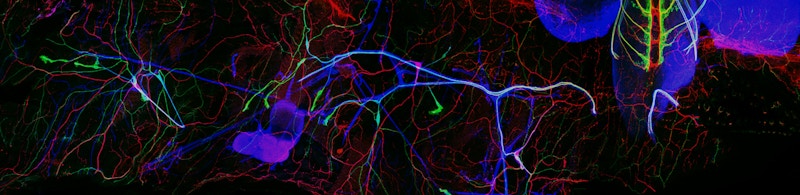
These findings were exciting, but because the flies in our experiment were stationary, they were not an exact replica of a fly’s behavior in the natural world. My next goal was to observe this behavior under a microscope in a freely moving fly.
In the real world, many animals, including flies, will navigate toward a good odor by walking or flying upwind since that is likely to lead them to the odor’s source. With help from Rockefeller’s microfabrication facility, I designed and built a sort of olfactory virtual reality for flies. We tethered the fly under a microscope and let it walk on a tiny air-supported ball, like a treadmill. As the fly walked, we could track the ball’s rotation and use that to adjust the direction of the wind blowing on the fly from a small air tube mounted on a specialized motor. This allowed the fly to reorient itself relative to the wind. In this virtual environment, we tracked whether a fly liked or disliked an odor based on whether it walked upwind when the odor was presented.
This system has since formed the basis for several follow-up studies in the lab where, in collaboration with other scientists in the Ruta lab, we were able to further investigate the circuitry underlying learning and context-dependent behavior in response to odors.
How has the Simons Junior Fellowship helped your journey?
Like many Junior Fellows have said, I value the intellectual ferment of the Fellowship and really enjoy our weekly dinners. The in-person dinners are best. Zoom ‘dinners’ during the pandemic were not quite the same, but at a time when we were all feeling pretty isolated, it was still great to be connected to the community. Besides those benefits, I have a specific reason to be grateful to the Simons Foundation. Right now, I’m in the third year as a Junior Fellow, which would normally be the final year. But very early in the pandemic, just as things were shutting down, the foundation announced that current Fellows could apply for a year of additional funding. Knowing this extension was possible in the spring of 2020 let me focus on taking care of my two young kids, as my wife was a medical resident on the front lines of treating COVID patients at the time. And it gave me the mental space to realize that I wanted to reach out to and teach undergraduates, and to work on diversity in science. This all happened because of the financial security provided by the foundation, which was truly life changing.