
In Aging Worms, a Window Into Cognitive Decline
The roundworm Caenorhabditis elegans thrives all over the world, in part because of its surprising capacity to learn. The tiny, undulating invertebrates are exquisitely tuned in to their surroundings, able to detect thousands of different odors, gauge salt and oxygen gradients, and register temperature shifts of a tenth of a degree. They use that information to stay safe and well fed.
Some sensory cues are inherently attractive or aversive for C. elegans, but many of their associations are learned through experience. In Coleen Murphy’s lab at Princeton University, researchers teach worms to associate the sweet, sharp smell of butanone (a neutral odor for untrained C. elegans) with the promise of food. Negative experiences, such as an encounter with pathogenic bacteria, can teach worms to avoid once-neutral stimuli. And inherently attractive signals, such as the scent of benzaldehyde, can lose their appeal when they are presented to worms in times of starvation. “They’re learning the whole time, no matter what you’re doing with them,” Murphy says.
Young worms take readily to these tasks, but as they age, their memory deteriorates — just as it does in humans. Murphy, who directs the Simons Foundation’s Collaboration on Plasticity and the Aging Brain, wants to know why. Studies in worms have already expanded researchers’ view of the molecules that contribute to memory and life span. And with their short life span, genetic tractability and simple nervous system, worms are a powerful model for investigating what changes during aging to cause cognitive decline.
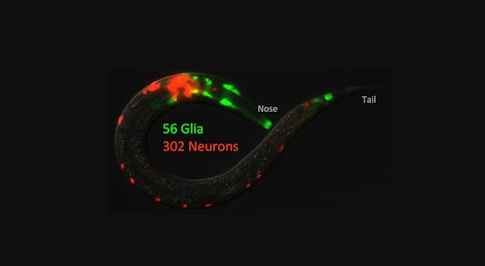
Because C. elegans uses many of the same molecules and mechanisms as humans to learn and remember, Murphy hopes that deciphering the causes of age-related cognitive decline in worms will reveal potential strategies for preserving cognitive function in people. “I think people don’t realize how well conserved evolutionarily these genes and molecules are,” she says. “If things are really analogous in neurons, then maybe [by] fixing the things that are broken [in worms], we’ll be able to find something that will be a good target in mammals.”
A valuable model
C. elegans has long been prized as a valuable model for aging research. With a life span of less than three weeks, the worms offer a more efficient means of studying age-related changes than most model organisms. Both genetic manipulation and caloric restriction can extend worms’ life spans, and researchers have used both as tools to investigate how and why aging impacts essential functions from motility to reproduction.
C. elegans is favored by many neuroscientists, too, in part because of its simple nervous system, which includes just 302 neurons and a comprehensively mapped connectome. Experiments on C. elegans, unlike studies of many model organisms used in neuroscience, can usually be done quickly and with large numbers of animals, says the neurobiologist Aakanksha Singhvi, who is using C. elegans to study glia in her lab at the Fred Hutchinson Cancer Research Center. “A thousand animals is not a big deal for me,” she says. “So that makes things much easier and simpler.”
A nearly complete wiring diagram has been available for C. elegans since 1986, when Sydney Brenner and colleagues traced the cells’ connections through serial electron micrographs. That diagram, together with developmental studies from Brenner’s lab, suggested that the worms’ nervous system was hard-wired, and most researchers assumed that it lacked plasticity. But in 1988, the community began to recognize worms’ capacity to learn when Catharine Rankin, a psychologist at the University of British Columbia, showed that their response to a mechanical stimulus changes after repeated exposure.
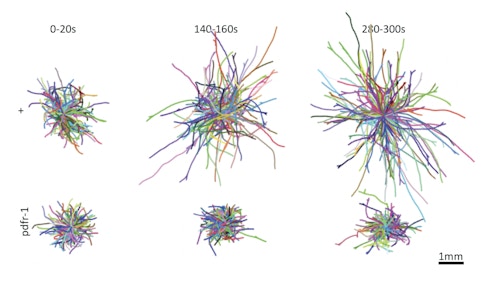
A simple tap on a dish of worms will initially provoke them to back away, but Rankin found that this response fades if the tapping continues. This habituation response allows worms and other organisms, including humans, to tune out repetitive stimuli, freeing up the nervous system to focus on more novel information.
Habituation is one of the simplest forms of learning, but Rankin says it’s more complex than it appears. “I think habituation is a superb assay of the health of a synapse,” she says. Measuring different components of habituation assesses both the efficiency of a synapse and how quickly it recovers from a stimulus. Her studies have uncovered how different molecular components on each side of the synapse shape worms’ weakening response to a recurring tap. She has also tracked how long it takes, once the repetitive stimulus stops, for worms to recover from habituation and resume their baseline response when tapping resumes.
Older worms don’t back up as much as younger worms in response to tapping, but they do habituate to tapping. When they do, it takes them longer to recover from that habituation once the tapping stops. It’s not clear to what extent these changes are due to learning deficits versus declines in motility or sensory processing, but Rankin says it’s easier to explore these questions in worms than in animals with more complex nervous systems.
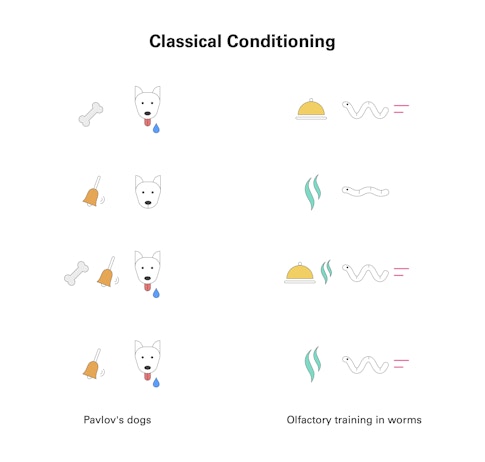
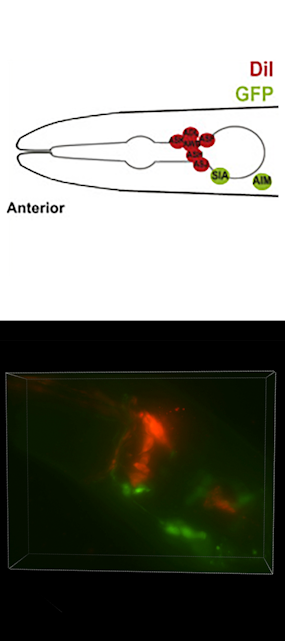
Complex learning
Habituation is a form of nonassociative learning, in which animals change their response to a stimulus without reinforcement. Worms are also capable of associative learning, a more sophisticated form of learning that links one stimulus to another through conditioning. Pavlov’s dogs learning to anticipate food at the ring of a bell is a canonical example. Murphy’s experiments teaching worms to like or dislike previously neutral odors are another.
By 2010, Murphy had demonstrated that C. elegans can form long-term olfactory memories, and that they use the same molecules and mechanisms that enable long-term memory in other animals. Once hungry young worms learn to associate the scent of butanone with a feast of nutritious bacteria, they migrate expectantly toward that scent for hours, hoping to find their next meal. A single training session is enough for a worm to form this kind of short-term olfactory memory. With repeated encounters to reinforce the link, the memory persists, guiding the worm’s explorations for a full day. This long-term memory requires protein synthesis, gene transcription, and the upregulation of a transcription factor called CREB, which is known to be critical for long-term memory in animals from sea slugs to humans.
At the University of Michigan, the neurobiologist Eleni Gourgou has shown that C. elegans worms are capable of yet another type of associative learning. With custom-designed mazes, her team found that young worms can return to a site where they previously found food by using tactile and proprioceptive cues to navigate the same route. Worms may not make the kind of mental maps that typically define spatial learning in higher organisms, Gourgou says, but they do remember how their own bodies have moved through and interacted with a space.
Middle-aged worms, however, fail to perform this type of learning. Her team has just begun investigating the neural circuits that underlie this behavior, but she says it could be a valuable new way of studying learning and how it is impaired both by normal aging and by disease. She imagines there may be hubs in the neural circuitry whose sensitivity to age-related changes causes multiple cognitive functions to deteriorate later in life.
While learning of all types fades over the few weeks of a worm’s life, the most complex learning is the most sensitive to age. Long-term memory is the first to go: By the time adult worms are five days old, the worm equivalent of middle age, they are unable to retain associative memories for more than a few hours. That makes sense from an evolutionary perspective, Murphy says, because worms stop producing eggs around this time, and finding food becomes less urgent. By day eight or nine, worms lose the ability to form short-term memories, but nonassociative learning and simple behaviors like chemotaxis continue even later in life.
When paired with a robust toolkit available for genetic manipulations, these behaviors make C. elegans an efficient system for interrogating the mechanisms behind age-related cognitive decline. Some structural changes have been noted in C. elegans’ neuronal projections by the time adults are eight days old. But the earliest memory impairments precede these morphological effects and may be due to subtle molecular changes that reduce the plasticity of neural circuits.
Murphy’s team has found that worms’ capacity for long-term memory correlates with levels of the CREB transcription factor, which naturally decline during aging. Now they’re examining the molecular consequences of this change. “CREB’s absolutely required for long-term memory, but it’s not the end of the road,” Murphy says. “It’s the thing that turns on everything else to allow memory to happen.” A genome-wide analysis published in 2015 identified more than 700 C. elegans genes that are induced by CREB during long-term memory training. Researchers tested the function of dozens of these genes using RNAi silencing and found that all of them were important for long-term associative memory. Some had already been implicated in memory in other organisms. Others, including many with mammalian counterparts, had not previously been tied to long-term memory, suggesting novel mechanisms yet to be explored.
Boosting memory
Though there are lots of ways to impair memory in worms, researchers have also found ways to improve it or delay its decline until later in life. Dietary restriction boosts both life span and some cognitive functions, as do some genetic manipulations. In Murphy’s lab, her then-graduate student Geneva Stein improved worms’ memory by introducing a hyperactive version of a protein called EGL-30. Wild-type worms require seven training sessions to create long-lasting olfactory memories. Worms with the hyperactive protein form long-lasting memories after just one.
The overactive protein can even restore memory in older worms when it is introduced later in life, according to work by Rachel Arey, a former postdoc in Murphy’s lab. It appears to do so by enhancing the activity of CREB, so even when the transcription factor’s level has dwindled, its target genes still get activated. Murphy is now collaborating with Saul Villeda of the University of California, San Francisco, a member of SCPAB’s executive committee, to investigate whether the same principles are at play in mammals, in hopes that manipulating this pathway might be a way to preserve memory in patients.
Arey, who is now studying C. elegans’ age-related cognitive decline in her own lab at Baylor College of Medicine, notes that over-activating the protein itself might not be safe: Mutations in the human homolog of the gene, a G-protein subunit called Gαq, can cause neuronal overexcitability and seizures. But understanding the protein’s downstream effects could help researchers identify potential drug targets.
Much of the research into cognitive aging in worms has focused on neurons, but Singhvi is using C. elegans to explore the role of often overlooked glial cells. “Most of the focus instinctively goes to thinking about neurons — neurons are degenerating, and neurons are in trouble,” she says. “But in my mind, that’s kind of half your brain. So you are looking at half the story.” (For more on the role of glia in aging, see “Are Similar Processes at Work in Both Brain Development and Cognitive Decline?”)
Singhvi’s team is building a molecular atlas that details the transcriptional profiles of each of C. elegans’ 56 glial cells in both one-day-old and eight-day-old adults. They will use the atlas to investigate how glia contribute to a variety of behaviors in C. elegans, including the worms’ ability to learn to associate particular temperatures with food, and what happens to glial interactions with neurons and other cells as the animals age.
Worms are a useful model for studying the neuron-glia interactions that support neuronal signaling because, unlike in higher organisms, each glial cell communicates with a predetermined neuron or set of neurons. Singhvi favors this more controlled experimental setting for dissecting exactly how glia communicate with neurons and influence their behavior. “You’re not looking at a pool of glial cells talking to a pool of neurons,” she says. “You can go very clearly into single-cell resolution and be very definitive about it.”
While Singhvi and other C. elegans researchers are confident that worms will provide important insights into the mechanisms behind cognitive aging, they know collaborations with scientists working in other systems will be essential to translating their findings into clinical advances. They want colleagues to know that worms offer unique opportunities to explore the factors that determine not just longevity, but also our quality of life as we age.
For Arey, who started her lab at Baylor in 2019, one of the most exciting aspects of her new role has been the opportunity to communicate with colleagues about C. elegans’ value as a neuroscience tool. “I try and go out and ask what can worms do for you?” she says. “Think about it. Ask me if they can do it. And I bet they can.”