New Material Pushes Limits of Superconductivity’s ‘Cousin’
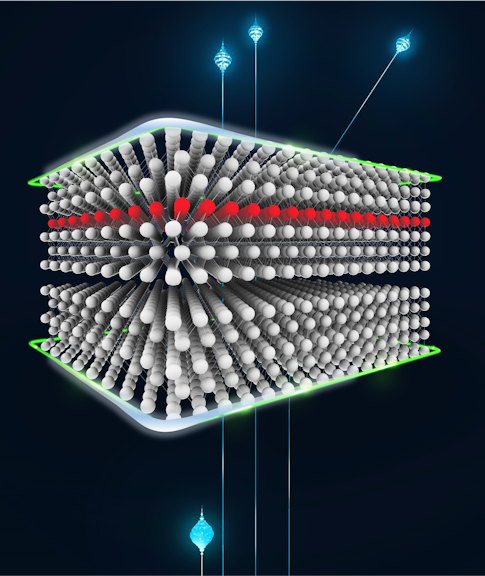
For decades, scientists have hunted for materials in which electrons flow without resistance. Until recently, this hunt focused squarely on superconductors, in which electrons pair off and flow freely. But superconductors aren’t the only game in town. In 2013, for the first time scientists observed a phenomenon known as the quantum anomalous Hall (QAH) effect. Just like in a superconductor, electrons under the QAH effect flow without dissipating energy, albeit via a different mechanism. Now a new material is pushing the QAH effect to new limits, dramatically increasing the temperature and conditions in which the effect occurs.
In a recent paper in Nature Physics, physicists presented a new material consisting of sandwiched layers of bismuth, tellurium and manganese. The material exhibits the QAH effect up to roughly 7 degrees Celsius above absolute zero, the research reports. That temperature is still much lower than the temperatures boasted by high-temperature superconductors, but it’s a giant leap forward from the 2 degrees above absolute zero previously shown for the QAH effect.
The new material edges the QAH effect toward viability in applications such as quantum computers, says study co-author Jennifer Cano, an affiliate associate research scientist at the Flatiron Institute’s Center for Computational Quantum Physics (CCQ) in New York City.
“There’s a future for applications, hopefully, but right now, no one is working on actual applications,” says Cano, who is also an assistant professor at Stony Brook University in New York. “Right now, we’re looking at what material combinations can we play around with and how far we can push this.” Cano worked on the new paper’s theoretical and computational side with Alexandru Georgescu, a Flatiron research fellow also at the CCQ.
While any applications remain far off, the potential use of QAH materials in quantum technologies such as error-free quantum computing serves as a “guiding light” for ongoing research, says study co-author Lia Krusin-Elbaum, a physics professor at the City College of New York. Krusin-Elbaum led the development of the material along with Haiming Deng, a doctoral student in her lab.
An electron highway
Understanding material properties such as the QAH effect comes down to understanding what electrons are doing. In a conducting material, electrons flow from high electric potential to low electric potential, like a rock rolling down a hill. The addition of a strong magnetic field twists the electrons, causing them to move perpendicularly to the ‘slope’ rather than downhill. This phenomenon is known as the Hall effect after Edwin Hall, who discovered it in 1879.
Looking at the Hall effect at the quantum level, you’ll find that the electrons move in discrete channels through the material. “Similar to how separated highway lanes allow for faster vehicle transit, the discrete channels allow for more efficient transfer of electrons,” says Georgescu. “The paths protect the electrons from smashing into other electrons and slowing down and losing energy.”
The need for a strong external magnetic field limits the utility of the Hall effect. But with the QAH effect, no external magnetic field is needed. Instead, the material provides its own magnetic field.
Early examples of the QAH effect were produced when researchers added magnetic impurities to a material known as a topological insulator. In these efforts, the QAH effect occurred — though the process required temperatures as low as 0.03 degrees above absolute zero.
In the new research presented in Nature Physics, the researchers took a more ordered approach. Rather than adding impurities to a material in a scattershot way, they carefully put down alternating layers of bismuth telluride — through which the electrons flow — and a manganese compound, which provides the magnetism. In addition to producing the QAH effect at higher temperatures than ever before, the new material is a metal rather than a topological insulator. This marks the first time a metal has been used to bring about the QAH effect, meaning the phenomenon can occur in more types of materials than previously demonstrated.
Altogether, the research suggests that the QAH effect can be pushed even further toward viability in electronics, Georgescu says. How far the limits can be pushed will be the subject of future research. For instance, one proposal suggests that adding an extra layer to a QAH material can produce the qubits used to crunch numbers in quantum computing.
“While we’re not looking at applications yet, the combination of increased temperature and the novelty of this effect happening in a metal opens up a wide array of new possibilities,” Georgescu says. “It’s exciting.”