Why Pulsars Shine Bright: A Half-Century-Old Mystery Solved
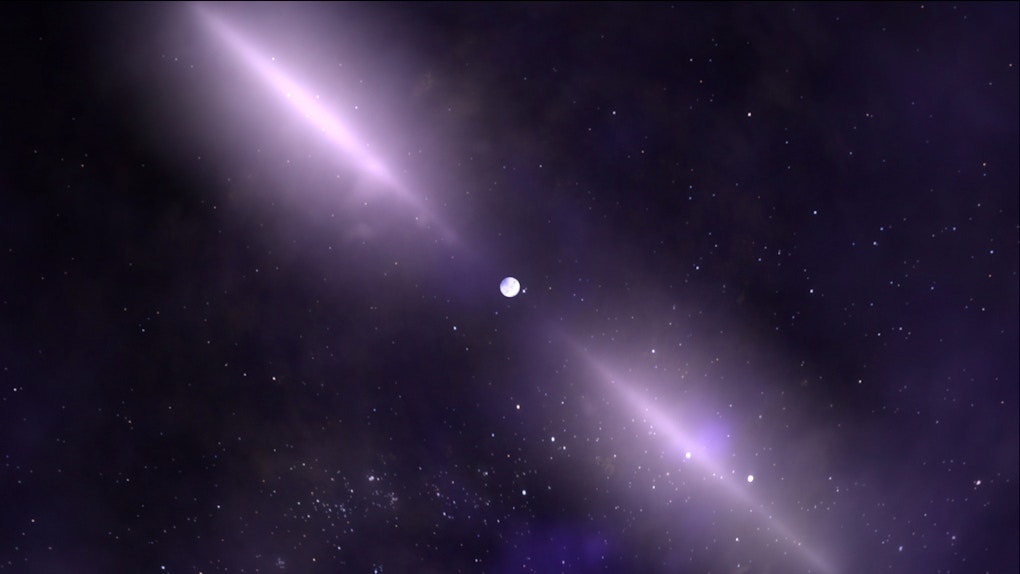
When Jocelyn Bell first observed the emissions of a pulsar in 1967, the rhythmic pulses of radio waves so confounded astronomers that they considered whether the light could be signals sent by an alien civilization.
The stars act like stellar lighthouses, shooting beams of radio waves from their magnetic poles. For more than a half-century, the cause of those beams has confounded scientists. Now a team of researchers suspects that they’ve finally identified the mechanism responsible. The discovery could aid projects that rely on the timing of pulsar emissions, such as studies of gravitational waves.
The researchers’ proposal starts with the pulsar’s strong electric fields, which tear electrons from the star’s surface and accelerate them to extreme energies. The accelerated electrons eventually begin emitting high-energy gamma rays. These gamma rays, when absorbed by the pulsar’s ultra-strong magnetic field, produce a deluge of additional electrons and their antimatter counterparts, positrons.
The newborn charged particles dampen the electric fields, causing them to oscillate. The wobbling electric fields in the presence of the pulsar’s powerful magnetic fields then result in electromagnetic waves that escape into space. Using plasma simulations, the researchers found that these electromagnetic waves match radio waves observed from pulsars.
“The process is a lot like lightning,” says study lead author Alexander Philippov, an associate research scientist at the Flatiron Institute’s Center for Computational Astrophysics in New York City. “Out of nowhere, you have a powerful discharge producing a cloud of electrons and positrons, and then, as an afterglow, there are electromagnetic waves.”
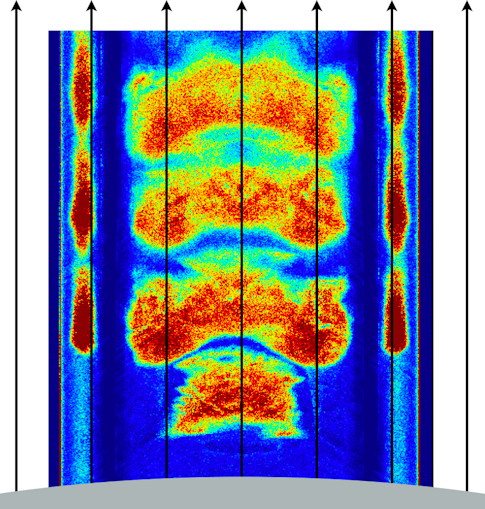
Philippov and collaborators Andrey Timokhin of the University of Zielona Góra in Poland and Anatoly Spitkovsky of Princeton University present their findings June 15 in Physical Review Letters.
Pulsars are neutron stars, the dense and highly magnetized remains of collapsed stars. Unlike other neutron stars, pulsars spin at dizzying speeds, with some rotating more than 700 times each second. That spinning generates powerful electric fields.
At a pulsar’s two magnetic poles, continuous beams of radio waves blast into space. These radio emissions are special in that they are coherent, meaning the particles creating them move in lockstep with one another. As the pulsar rotates, the beams sweep in circles across the sky. From Earth, pulsars appear to blink as the beams move in and out of our line of sight. The timing of these blinks is so precise that they rival the accuracy of atomic clocks.
For decades, astronomers pondered the origins of these beams but failed to produce a viable explanation. Philippov, Timokhin and Spitkovsky took a fresh approach to the problem by creating 2D simulations of the plasma surrounding a pulsar’s magnetic poles (previous simulations were only 1D, which can’t show electromagnetic waves).
Their simulations replicate how a pulsar’s electric fields accelerate charged particles. That acceleration produces high-energy photons that interact with the pulsar’s intense magnetic field to produce electron-positron pairs, which are then accelerated by the electric fields and create even more photons. This runaway process ultimately fills the region with electron-positron pairs.
In the simulations, the electron-positron pairs create their own electric fields that oppose and dampen the initial electric field. Eventually, the original electric field becomes so weak that it reaches zero and begins oscillating between negative and positive values. That oscillating electric field, if not exactly aligned to the pulsar’s strong magnetic field, produces electromagnetic radiation.
The researchers plan to scale up their simulations to get closer to the real-world physics of a pulsar and further probe how the process works. Philippov hopes that their work will ultimately improve research that relies on precisely observing the timing of pulsar emissions reaching Earth. Gravitational wave astronomers, for instance, measure tiny fluctuations in pulsar timing to detect gravitational waves stretching and compressing the fabric of space-time.
“If you understand how the emission itself is produced, there’s a hope that we can also produce a model of the errors in the pulsar clock that can be used to improve pulsar timing arrays,” Philippov says. Additionally, such a deeper understanding could help resolve the mysterious source of periodic bursts of radio waves, known as fast radio bursts, that emanate from neutron stars, he says.
The research was supported by NASA grant 80NSSC18K1099 and by the National Science Foundation under grants NSF AST-1616632 and NSF PHY-1748958.
Information for Press
For more information, please contact Stacey Greenebaum at [email protected].