How the Brain Holds On to Working Memory
When an animal holds an idea or plan in its working memory, the neurons that represent that idea can remain active for several seconds. But neurons usually react on a much shorter timescale: Inputs to a cell generally only affect its output for about 20 milliseconds. What happens during working memory that makes neurons capable of such sustained activity?
In research published in Nature in February, first author Hidehiko Inagaki, Karel Svoboda, an investigator with the Simons Collaboration on the Global Brain, and collaborators at the Janelia Research Campus explored how working memory functions in the mouse anterior lateral motor cortex. Specifically, they tested two different classes of hypotheses: cell-autonomous and attractor-based models. Cell-autonomous mechanisms involve a change in the internal state of a neuron that keeps its activity alive for longer than usual. Attractor mechanisms, on the other hand, require an entire network of neurons that provide input to each other to sustain a pattern of activity.
To distinguish between the two mechanisms, researchers recorded neural activity as mice performed a delayed motor task. First, a sound indicated whether the animal should lick to the right or to the left. Then the mouse had to wait for a short time before actually licking. The delay forced the mouse to remember its choice.
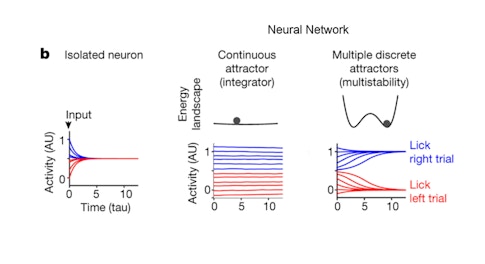
One way for a cell to keep firing during the delay period would be to alter the cellular machinery that converts neural input into output, either slowing it down or making the cell fire in extended bursts. But researchers saw no evidence of this. Whole-cell recordings that monitor the subthreshold activity of a neuron, combined with injection of current, showed no slowing of the cell’s internal dynamics and no change in the number of spike bursts during the delay period. This suggests that cell-autonomous mechanisms aren’t at play.
Turning to attractor mechanisms, the researchers analyzed neural activity in groups of cells during the delay period. Attractor mechanisms fall into two basic categories: discrete and continuous. In the former, the network can sustain only specific, separate patterns of activity, such as one for leftward licking and one for rightward. In the latter, activity can vary smoothly between these different patterns.
Because a continuous attractor can sustain activity patterns that differ from the strictly leftward or rightward states, it’s possible for the neural activity to wander away from these states as time goes on. If it wandered in different ways on different trials, neural variability would increase across trials over time. Instead, the researchers found a decrease in neural variability during the delay, suggesting continuous attractors are not at play. Furthermore, when researchers perturbed the network by optogenetically stimulating neurons, the activity snapped back to the rightward or leftward pattern, suggesting the presence of two discrete attractors. The researchers were also able to build a computational model of the circuit with two attractor states that accounted for much of the observed properties in the data.
The discovery that the anterior lateral motor cortex behaves like a discrete attractor has implications for the connections between the neurons in this area; only certain patterns of connectivity can support discrete attractors. This study thus demonstrates how measures of neural activity can inform our understanding of connectivity.