How Did the First Supermassive Black Holes Form?

Far, far away, at the edge of the observable universe, lurks the mystery of black holes that are millions to billions of times the mass of the sun. Equally monstrous black holes exist at the centers of galaxies, including our own Milky Way, because they have had billions of years to scarf down plenty of gas and dust. But how is it possible for black holes to do the same at the edge of the universe, in the early the universe, where there is comparatively little matter to feed them — and little time (less than 1 billion years) for them to consume it?
These are questions that Kohei Inayoshi, a theoretical astrophysicist at Columbia University and a Junior Fellow in the Simons Society of Fellows, is working to answer. He is also looking at the connection between the first stars in the universe, the relic radiation of the Big Bang, and gravitational waves. Inayoshi recently spoke with me about his work; an edited version of the interview follows.
What ideas do astrophysicists have for how supermassive black holes formed in the early universe?
One way black holes form is through the explosions of massive stars. If a star with a mass more than 30 times the mass of the sun stops fusing atoms in its core to make energy, the core can collapse, the star can explode and a black hole forms. My research focuses on creating scenarios where a black hole could grow very rapidly in the very early universe. We have to think of very crazy stuff, such as stars with 100,000 times the mass of the sun. We call them supermassive stars.
How might these supermassive stars have formed?
In star formation, you have a cloud of gas that’s dominated by its own gravity. The cloud collapses and stars form. Usually the gas clouds first fragment into smaller clouds, which then collapse into stars, which is why we have stars smaller than the sun and some that are 1,000 times as large. But to get really supermassive stars, the cloud can’t fragment. The only way to suppress fragmentation is to keep the temperature of the gas clouds high. If the temperature stays high, and the cloud tries to collapse, all the matter collapses into one star. Only in the early universe do we find some very specific conditions where this kind of collapse could happen. My Ph.D. project was to build a simulation to show how this might have happened.
What are the specific conditions that may have allowed these supermassive stars to form?
In the early universe, just after the Big Bang, we only had hydrogen and helium. All the other elements, such as carbon and oxygen, are produced by the nuclear fusion in stars, so they didn’t exist until after the first stars exploded and died. Hydrogen is very important as a cooling mechanism. We understand the microphysics of hydrogen, so we can estimate the cooling efficiency of hydrogen atoms and hydrogen molecules. Astrophysicists believe that the first stars, what we call population III stars, formed when hydrogen atoms combined to form molecules, which cooled the gas cloud. This molecular-hydrogen cooling forced fragmentation of gas clouds. That fragmentation is how you would get population III stars of 10 to 100 solar masses. To get the supermassive stars we propose, you need to suppress molecular-hydrogen cooling. To do this, you need a source of radiation from outside the cloud, possibly from other stars forming nearby or in galaxies nearby.
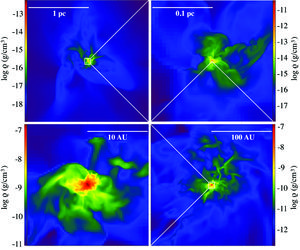
Do you think we will ever be able to detect these supermassive stars?
Yes, we think that the supermassive stars generated in our model should be detectable with the James Webb Space Telescope and other observational facilities of the future.
How do these supermassive stars collapse into supermassive black holes?
A protostar starts to condense at the center of these gas clouds. This protostar, which can start at about the size of the sun, can accrete up to one or two solar masses per year. Once it exceeds 100,000 solar masses, it collapses into a supermassive black hole just like any other massive star would.
Would there be observable evidence for these black holes?
Yes. Already astronomers have two candidates for these black holes, which we call direct-collapse black holes. And there may be other evidence in the future. Like supermassive black holes in the local universe, those that formed early on can affect stars growing nearby. When a star gets too close to a black hole’s event horizon, it’s pulled apart by tidal forces. We recently did calculations to show that stars that formed in the nuclear accretion disk of a direct-collapse black hole could experience tidal disruption, and those events could be observed with future X-ray detectors, such as eRosita. The James Webb telescope may also be able to probe feedback from direct-collapse black holes.
In a recent paper, you describe a gravitational wave background from population III binary black holes. How would this gravitational wave background form?
Gravitational waves are big right now, after the detection of black hole mergers by LIGO, the Laser Interferometer Gravitational-Wave Observatory. There are different sources for gravitational waves. Besides black hole mergers, gravitational waves can come from massive individual spinning objects. And then there are stochastic gravitational waves, which are passing by from all over the universe all the time. They are small signals that are hard to detect. Stochastic gravitational waves could come from black hole mergers that haven’t been detected yet. One promising source of these gravitational waves is the mergers of binary black holes that formed from population III stars born early in the universe.
How soon might researchers be able to detect these gravitational waves from black holes that formed from the first stars?
The recent calculations we did using data from the Planck space observatory suggest that population III binary black holes would create a gravitational wave background that would dominate in the 10-100 hertz range. It would dominate over other binary black hole populations. That means the gravitational wave background from mergers of population III binary black holes may be detectable sooner than we expected, maybe in the next five years.
The Simons Society of Fellows is a community of scholars that encourages intellectual interactions across disciplines and across research centers in the New York City area.