James Heys, Ph.D.
Northwestern University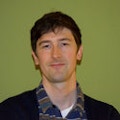
James Heys was a postdoctoral fellow in the laboratory of Daniel Dombeck at Northwestern University and is now an assistant professor of neurobiology at the University of Utah. Heys’ research uses a combination of in vivo cellular-level optical imaging, in vitro single-cell electrophysiology and biophysical modeling to understand the neurobiological substrates of spatial navigation and spatial memory. He received a Ph.D. in 2013 working with Michael Hasselmo at Boston University.
Project: Optical Imaging and Manipulation of Medial Entorhinal Cortex Grid Cells
Any animal, humans included, must be able to navigate and remember aspects of the environment in order to survive. For example, a squirrel must remember where it buried each of its nuts, or a mouse might need to remember where cats live so it can avoid them. But how does the brain represent space and how is this representation used to form memories? One area of the brain, termed the medial entorhinal cortex, or MEC, is known to be involved in spatial navigation and memory. In fact, specific neurons in the MEC are thought to contribute to spatial navigation skills. These cells are termed “grid cells,” which are called that because they are active in a grid pattern as an animal traverses an environment. For example, when you walk across the room, a grid cell might fire every few feet, creating a map of the space that you are in. It’s thought that the MEC and other brain regions use these cells to navigate environments and form memories about them. However, it’s unknown how these grid cells get their interesting properties. That is, how do neural circuits function in such a way as to produce cells that fire regularly in a grid? There are many theories, but these theories have been difficult to test because of technical limitations. We have developed a new way to observe the activity of grid cells while a mouse navigates a virtual reality environment. While much research has looked at grid cells one at a time, we will use sophisticated genetic and optical techniques to visualize the activity of hundreds of these grid cells at once while the animal moves about its virtual surroundings. In this way we can test which of the many theories of grid cell formation is likely to be correct. Our results will pave the way for understanding not only how the MEC uses grid cells to navigate, but will also offer more general insights into how neural circuits can shape the activity of individual neurons.