Making Electrons Behave In New and Unexpected Ways
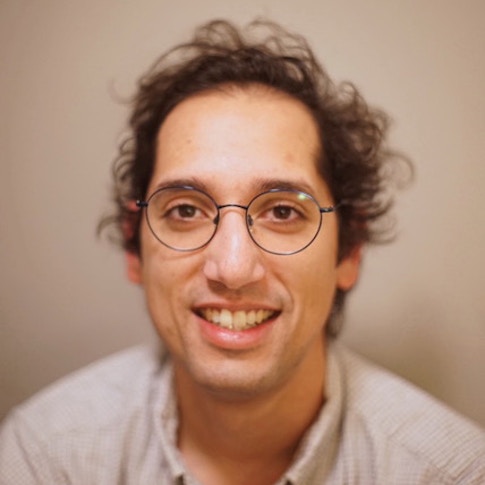
In high school, most students learn the basics about electrons: They are part of atoms, have a negative charge and carry electricity through solids. For many of us, that’s all we ever learn. But Aravind Devarakonda, who developed an interest in the behavior of electrons at his science-focused high school, discovered that there was much more to the story.
Devarakonda’s curiosity about electrons was first piqued when he learned about the electrical properties of silicon, the core component of computer chips. Thanks to that initial fascination, Devarakonda now creates materials that have unexpected electrical properties. His goal is to discover new electronic states of matter by using the tools of physics and chemistry.
Devarakonda recently completed his first year as a Junior Fellow with the Simons Society of Fellows at Columbia University, where he is studying with physicist Cory Dean. We recently discussed Devarakonda’s work and its implications. Our conversation has been edited for clarity.
How would you describe your overall scientific interests?
I’m drawn to the idea of ‘emergence,’ the scientific phenomenon that the whole is greater than the sum of its parts. There are many examples of this in nature. For example, in complex biological systems, individual components can often come together to act in unexpected ways. In my research, I focus on how large numbers of electrons move through solids, which can result in behaviors that are both surprising and emergent.
For me this interest started in a high school electrical engineering course, where we would tinker with electronics to build simple tools like clocks and thermometers. We were essentially manipulating the flow of electrons through the silicon inside computer chips. I was curious about how this process works, which led me down the rabbit hole of trying to understand how electrons behave in other materials — where I still find myself today, albeit with new and more novel structures. Once we better understand the properties of electrons in these materials, I’m hopeful that we can make them behave in new and interesting ways.
What’s a potential application of this work?
Typical superconductors — materials capable of conducting electricity without any resistance, like elemental aluminum and the compound niobium disulfide (NbS2) — superconduct at temperatures a few degrees above absolute zero. This makes them expensive and often impractical to develop into technologies. With a better understanding of how electrons behave in solids, perhaps we can develop new superconductors that are much more practical to operate. That may be decades in the future, but I don’t think it’s out of the question.

My work is curiosity-driven. The materials that I synthesize and study might not have immediate applications, but I’m sure that the understanding I’m bringing with my research will help pave the way toward useful tools in the long run.
One particularly promising type of material is called a topological insulator, which is unusual in that it conducts electricity on its surface but not in its interior. Topological insulators and other topological materials have attracted a lot of attention over the past two decades, spurred in large part by several key theoretical insights.
Can you talk more about those insights?
Approximately 20 years ago, physicists Charles Kane, Eugene Mele and Shoucheng Zhang, and their research groups, identified key information about the fundamental behavior of electrons in crystals. Specifically, they found that electron wave functions in crystals can be grouped into topologically distinct classes, meaning they cannot be transformed into each other without an accompanying drastic change in their properties. This would be like trying to change a sphere into a donut without punching out a big hole in the middle.
Several years after this initial prediction, experimentalists demonstrated the existence of these topological materials. Since then, our community has worked to catalog different kinds of these materials. More broadly, this approach to examining the physics and chemistry underlying materials is a crucial step toward potential future applications, such as building new types of superconductors.
What was the main focus of your doctoral work at MIT?
My research centered on a type of material called a superlattice. A superlattice is a structural arrangement created by stacking two or more distinct layers one on top of another, sort of like a layer cake. Superlattices can come in many varieties. My focus was on a particular superlattice, barium niobium sulfide.
Finding this superlattice was, in a way, the result of a happy accident related to another project I was pursuing. I was trying to synthesize a compound composed of the elements barium, niobium and sulfur. It had been predicted that this compound would behave like a three-dimensional analog of the single carbon layer known as graphene. During my experiments, I noticed that some of the crystals obtained were shiny and layered. As we tried to understand its structure, we found it to be a really intriguing superlattice.
The superlattice we grew is related to a larger family of materials known as transition metal dichalcogenides, or TMDs. In our superlattice, individual layers of the superconducting TMD niobium disulfide are isolated from each other by an insulating block. At low temperatures, this TMD also conducts electricity with zero resistance. In essence, it was a three-dimensional crystal that behaved as if it were a two-dimensional superconductor. It is also a very clean material, meaning there aren’t many defects in the structure.
When we looked a bit more closely, we found our material to exhibit an unusual type of superconducting state that normally only appears when a material is both exceptionally clean and two-dimension-like. And bringing it full circle, it turns out this material also appears to be topological, similar to the compound I initially set out to study. This discovery, and others like it, expand our understanding of how superconductivity and topological properties manifest in real materials. With this knowledge, we could potentially grow new materials where these electronic properties are more robust, for example, surviving to higher temperatures.
Now that you’re at Columbia, how have your research interests evolved?
In graduate school, I had a top-down approach, by growing a macroscopic crystal and studying its properties. Now, at Columbia, my work is more bottom-up, such as stacking sheets of a material like graphene together, to create artificial structures that we can manipulate and use to study the behavior of electrons. Both approaches have the same goal, which is realizing new electronic states of matter.
At Columbia, I work closely with chemistry colleagues in the Roy Lab. Traditionally, there have been walls dividing physics from chemistry. It is my hope that my work, and the work of my colleagues, is helping to break down that barrier — which is very exciting.
Indeed, my work lies at the intersection of chemistry and physics, as applied to studying unusual electronic phases of matter. I might discover a new insight about the behavior of electrons in a crystal that would feed back into the chemistry ‘recipe’ I used to grow the crystals, thereby giving us the ability to potentially manipulate their properties.
Finally, what are your thoughts about the Simons Junior Fellowship?
The interaction between the Junior and Senior Fellows is a wonderful and unique experience, because in a way it breaks down the formal barrier between faculty and postdoc. It’s invaluable to be able to have conversations with the Senior Fellows in a casual, open environment during our weekly dinners and lectures.
But more fundamentally, the Simons Foundation has a strong history in supporting research into complex systems with emergent behavior, of the kind I study, so it’s a perfect place to be. I sometimes find inspiration from how scientists in other related fields make progress toward understanding other complex systems, so I’m very grateful for this community.