Decoding How We Make Quick Decisions
Simons Junior Fellow Michael Waskom studies what happens in the brain when we need to decide something quickly, like whether to cross the street against a red light. To do this, Waskom marries granular data about how individual neurons operate to an overarching theory about how the brain as a whole drives decision-making.
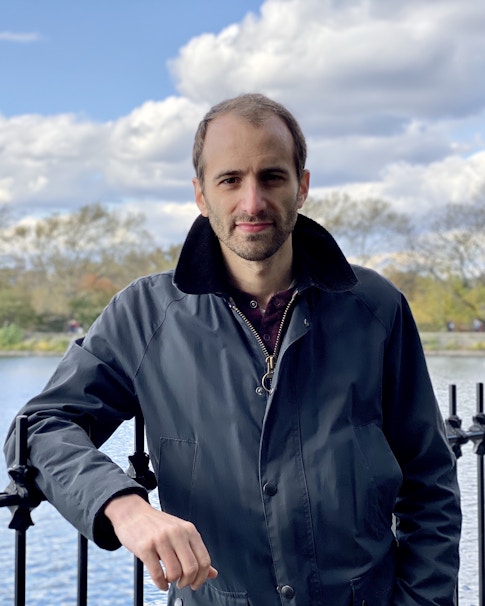
People often think of the brain as a patchwork quilt of regions, each with a different function. According to Michael Waskom, a Simons Society of Fellows Junior Fellow and a postdoc at New York University’s Center for Neural Science, this notion is too simple: It fails to explain how different brain regions work collectively to make hundreds or even thousands of decisions throughout the day.
Waskom, whose adviser is Roozbeh Kiani, discussed his ongoing research and what he has valued during his Simons fellowship. Our conversation has been edited for clarity.
How would you describe your core research question?
Broadly, I am seeking to understand the underlying brain mechanisms of behavior, especially how the brain makes decisions. It’s easy to come up with different kinds of explanations for something as complex and ubiquitous as decision-making. For example, you might introduce a computational theory of the factors that matter and how we weigh them when making a choice. Or you could describe the pattern of neural activity that can be measured in an experiment. Both approaches are important. You need neural data to constrain the computational theories, and to help push them in new directions. But without theories to guide interpretation, neural data rarely make sense on their own. The best explanations operate at both levels: They give a computational account of brain processes that can then be observed in neural data.
Can you drill down into exactly which brain processes you study?
Every animal — from the tiny fruit fly to the enormous blue whale — has a set of behaviors that range between purely reflexive actions and those that are more flexible and goal-directed. For example, putting your hand on a hot stove and immediately pulling it away is reflexive — that’s the spinal cord in action, not really the brain.
But other kinds of actions, even relatively simple ones, are different. Let’s say you’re at a crosswalk and the ‘don’t walk’ sign is active. Do you cross? Your answer should consider several factors, such as distance to the nearest car, and how fast that car is moving. But it will also depend on your goals: You’d be more likely to cross if you’re running late for a meeting. I try to understand the mechanisms that integrate these different pieces of information to make these sorts of goal-directed choices.
At this point in the field, we understand basic reflexes well. We also have good models of some of the more flexible types of decisions, especially ones that require only a couple of seconds. Interestingly, in the brain these relatively quick decisions don’t look like snap judgments; both computational theory and neural data suggest that they result from a gradual, seemingly deliberative process. But of course, many decisions take even longer to play out. Part of my work has examined whether existing theories can account for decisions that feel more consciously deliberative. And another line of research looks at what happens when we need to focus on — or ignore — different kinds of information as we deliberate.
How has the overall scientific view of how we use our brain to make decisions evolved?
The classic view of the brain is that every region has a distinct role. So, the parietal cortex is involved in attention, while the prefrontal cortex makes decisions. This model has seeped into the popular consciousness. I’m sure you’ve heard people reference ‘the part of the brain that does X.’
But in recent years, some neuroscientists have turned away from that view. We now recognize that different regions generally don’t have unique roles, but instead work together in functional networks. Thinking about how information flows through these networks, rather than trying to identify the role of individual regions, can really change the way you approach neural data and our understanding of the brain.
What experiments have you developed to study decision-making?
I aim for tight experimental control, which allows me to develop detailed computational models. To accomplish this, I typically borrow tasks from sensory neuroscience, where such control is also paramount. This has a cost, because the tasks are often rather artificial and not a direct reflection of real-world decision-making. But it also lets me incorporate our existing understanding of the brain’s sensory systems, which reduces the unknowns in my models.
One such experiment is called the random dot motion task, which asks you to view many small dots as they move around a computer screen in different directions. Some percentage of the dots will be programmed to move in one particular direction, and I’ll ask you to decide which direction that is: say, left or right. By varying the percentage of dots that move together, I can control the difficulty of the decision in a very precise way.
A variation of this experiment would be to assign each dot one of two colors, like red or green, and again program some percentage to appear as one color or the other. Sometimes I would ask you to focus on the motion of the dots, and other times I’d ask you about the color. This lets me study how your brain flexibly changes your decision strategy. We do this all the time in real life. Think back to the example of deciding whether to cross against the light. When that’s your goal, you should pay attention to how fast the cars are moving. But if your goal is to hail a taxi, it would be more important to focus on their colors.
Are there any surprising findings from your research?
Yes, although whether you’re surprised might depend on whether you think of decisions as something that people make, or as something that neurons make.
I’ve found that the same computational theory that explains how we make quick, almost split-second decisions can also account for decisions that take much longer, say up to half a minute. In both cases, we can continually accumulate information to make better choices. Now on the one hand, half a minute doesn’t seem like a very long time. We certainly take longer to make up our minds about weighty decisions, such as whether to accept a job offer. But individual neurons act much faster than that. They’re only ever responding to inputs that they received in the past few milliseconds. A big question has been: How can you wire a bunch of neurons together to make a network that holds information for longer durations? We can build models to explain how the brain does that for quick decisions, but even the best models start to ‘leak’ information after a few seconds. So my results suggest that standard models are missing some key mechanism.
Sounds like further work awaits! I also noticed that you design open-source software for displaying research statistics elegantly. Please talk a bit about that.
Sure. It’s a data visualization library called seaborn, which I started when I was completing my Ph.D. I started it so that I could make my own figures better, but it’s caught on, and now it’s used pretty widely. It’s written in the programming language Python, which, like seaborn itself, is open source. This means anyone can download it for free and use it. I’ll go to conferences and see posters by people I’ve never met who are using it to make their graphs. That’s very cool!
It’s always gratifying when people value something we create! Let’s end with your thoughts on your Simons fellowship, which is coming to a close after three years.
The fellowship is truly unique. Most of academia is siloed — sometimes you don’t even know what people right down the hall are doing, much less in another department. But the Junior Fellows span many fields, and the weekly dinners arranged by the Simons Foundation encourage us to learn from each other and to sharpen how we present our own research to people who are not in our field. It’s the very opposite of a silo! And I am grateful for the opportunity.